Unusual Labmates: Bats
Super-bats
Bats are the only mammals capable of flight, and one of few mammals capable of using echolocation. They have captured people’s imaginations for centuries — as vampires, Halloween mascots, caped crusaders, and more — but more recently, bats have captured the attention of a growing number of biomedical researchers.
Bats have fascinating biological properties. These include resistance to cancer; long life spans relative to their body size; extreme cold tolerance; and tolerance of viruses: they carry many viruses harmful to humans, and yet often suffer few if any symptoms. In spite of these fascinating characteristics, bats have not been used in much biomedical research, because they are not easy to keep or study in the lab.
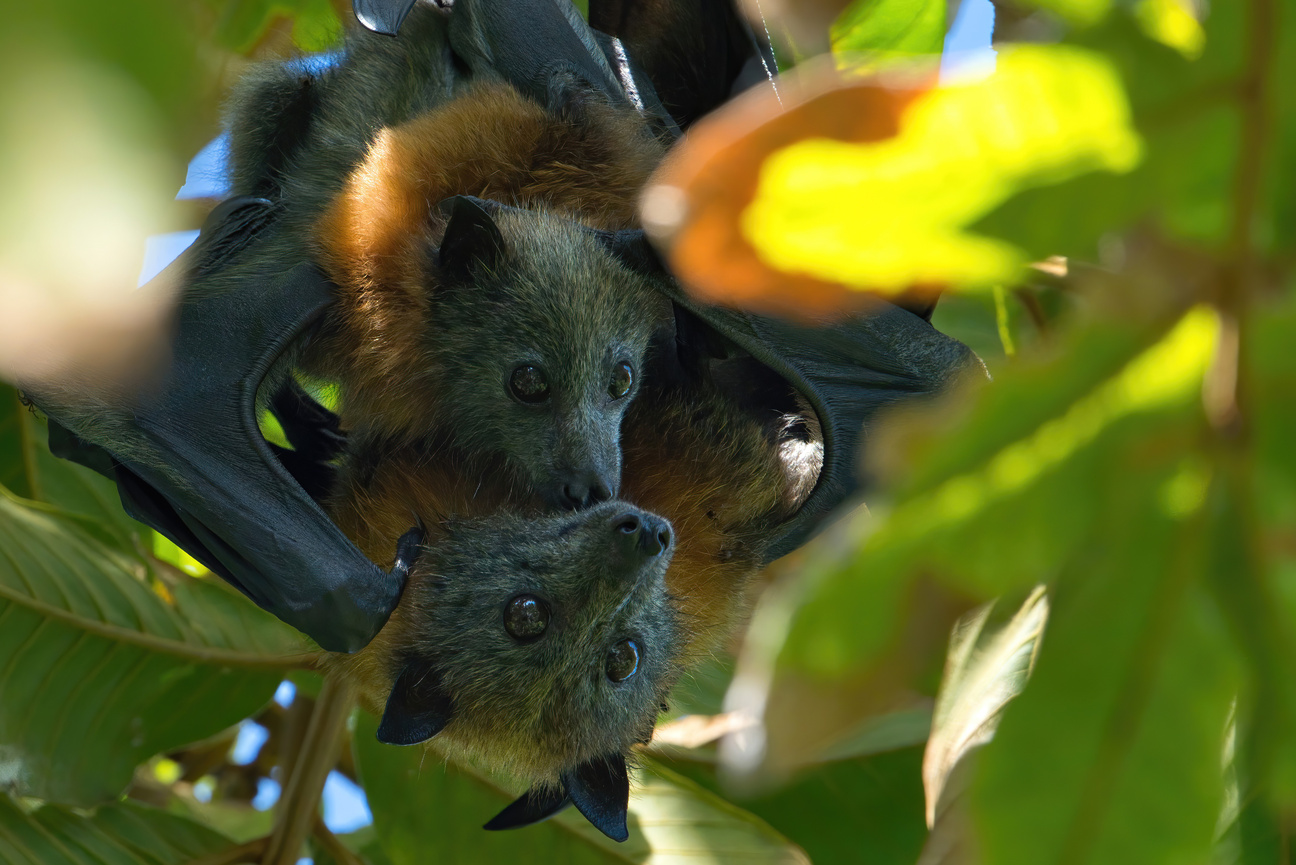
A grey headed flying fox (Pteropus poliocephalus) and its pup.
"Grey headed flying fox with pup - AndrewMercer - DSC10055" by Andrew Mercer is licensed under CC BY-SA 4.0.
However, interest in bats’ unusual biology surged during the early days of the COVID-19 pandemic. Bats carry a close relative of SARS-CoV-2, the virus that causes COVID-19 — they may even have been the new coronavirus’ original hosts — but are much better at avoiding severe symptoms and long Covid. As researchers around the globe raced to understand the biology of the new virus that had thrown the world into chaos, the value that bats could provide to research had never been more clear.
Read on to learn more about how an innovation made studying bat biology in depth possible, and how researchers at Whitehead Institute have made use of bat biology to study questions about viral infection, immunity, cold tolerance, and more. You can scroll or jump between chapters using the chapter bar at the bottom of the page.
How to study bat biology without any bats
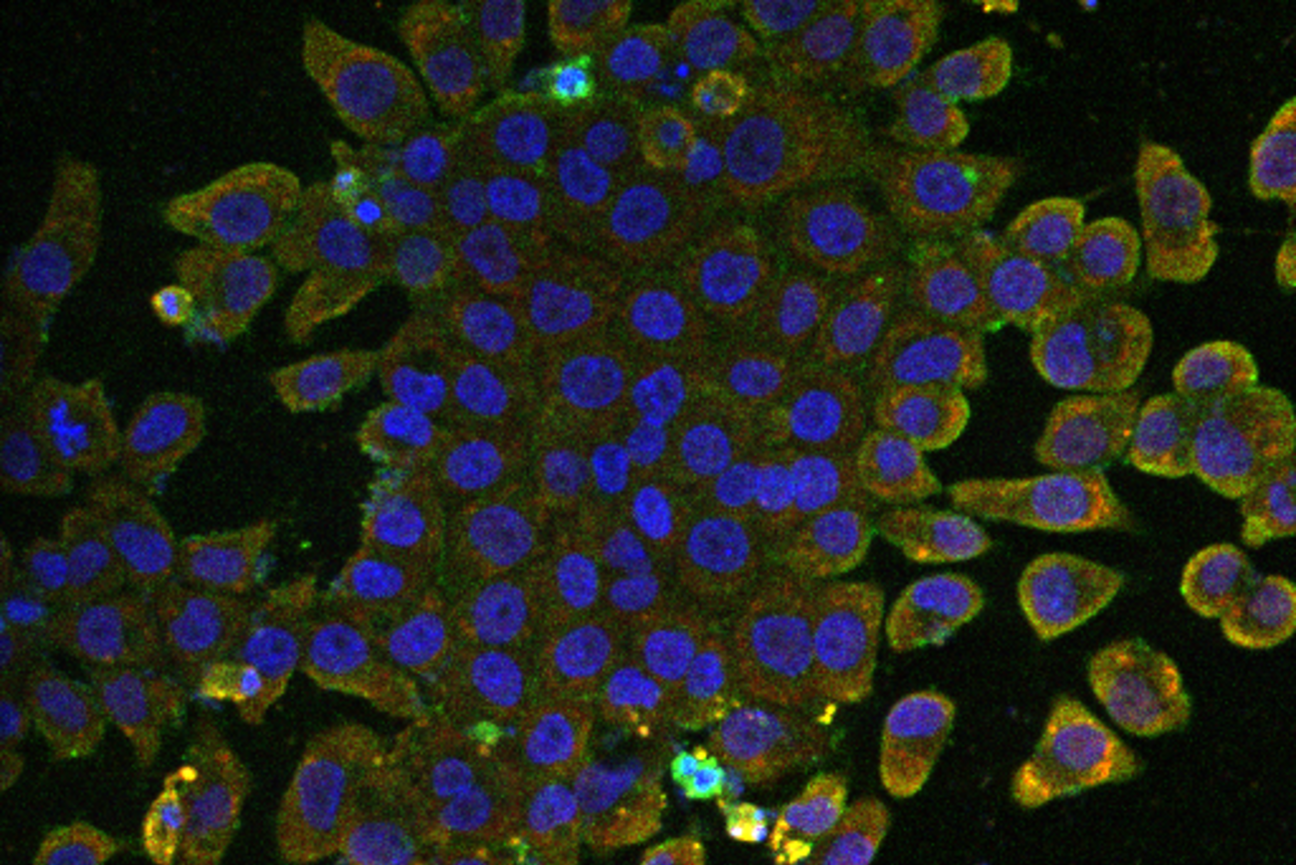
Induced pluripotent stem cells (nuclei in blue) from the bat species Rhinolophus ferrumequinum, which have been infected with SARS CoV-2 (marker in red).
Punam Bisht/Whitehead Institute
Bats have rarely been used in biomedical research because they are difficult to keep in captivity. Not only do they need lots of space to roost and fly, they also carry diseases that can transfer to humans, meaning that researchers can only interact with them using costly and time-consuming biohazard safety protocols. Their life cycle is also not conducive to research. For example, bats are slow to reach sexual maturity and have low numbers of offspring. Popular model organisms reproduce quickly, so that researchers can observe or engineer changes from one generation to another.
These factors all make bats, as animals, difficult to keep in a research laboratory. How, then, to study their fascinating biology? In the early days of the COVID-19 pandemic, Thomas Zwaka, a professor at the Icahn School of Medicine and Mount Sinai in New York, became interested in how bat biology could inform our understanding of viruses including COVID-19. Zwaka did not have experience with bats, but he was an expert at creating stem cell lines. Stem cells are pluripotent, meaning they can become (or be used to create) skin cells, blood cells, brain cells, and almost any other adult cell type. When a stem cell divides to make two new cells, typically one will be a new cell type and one will be another stem cell, so a lineage of stem cells can continue to divide and produce new cells indefinitely. Much of the current research done on human biology is in fact done using human stem cells, or cell types derived from human stem cells.
However, creating a new stem cell line, especially from a new species, is not a simple task. Researchers must cause adult cells to turn back into stem cells, which requires figuring out an exact recipe of signals and timing with which to trigger the adult cells. The researchers who figured out the recipe to turn adult human cells into a pluripotent stem cell line won a Nobel Prize for their efforts. Before 2020, no one who had attempted to create a bat stem cell line had succeeded.
In order to learn more about how bats tolerate SARS-CoV-2, Zwaka and colleagues took on the challenge. They applied their stem cell expertise to identify the right recipe to transform connective tissue cells taken from greater horseshoe bats (Rhinolophus ferrumequinum) back into stem cells. They used some of the same ingredients that are used to create human stem cell lines – a testament to how similar our biology really is, Zwaka says. The development of bat stem cell lines should make bat biology much more accessible to researchers, who no longer have to work with live animals in order to study what makes bats (and their cells) so resilient.
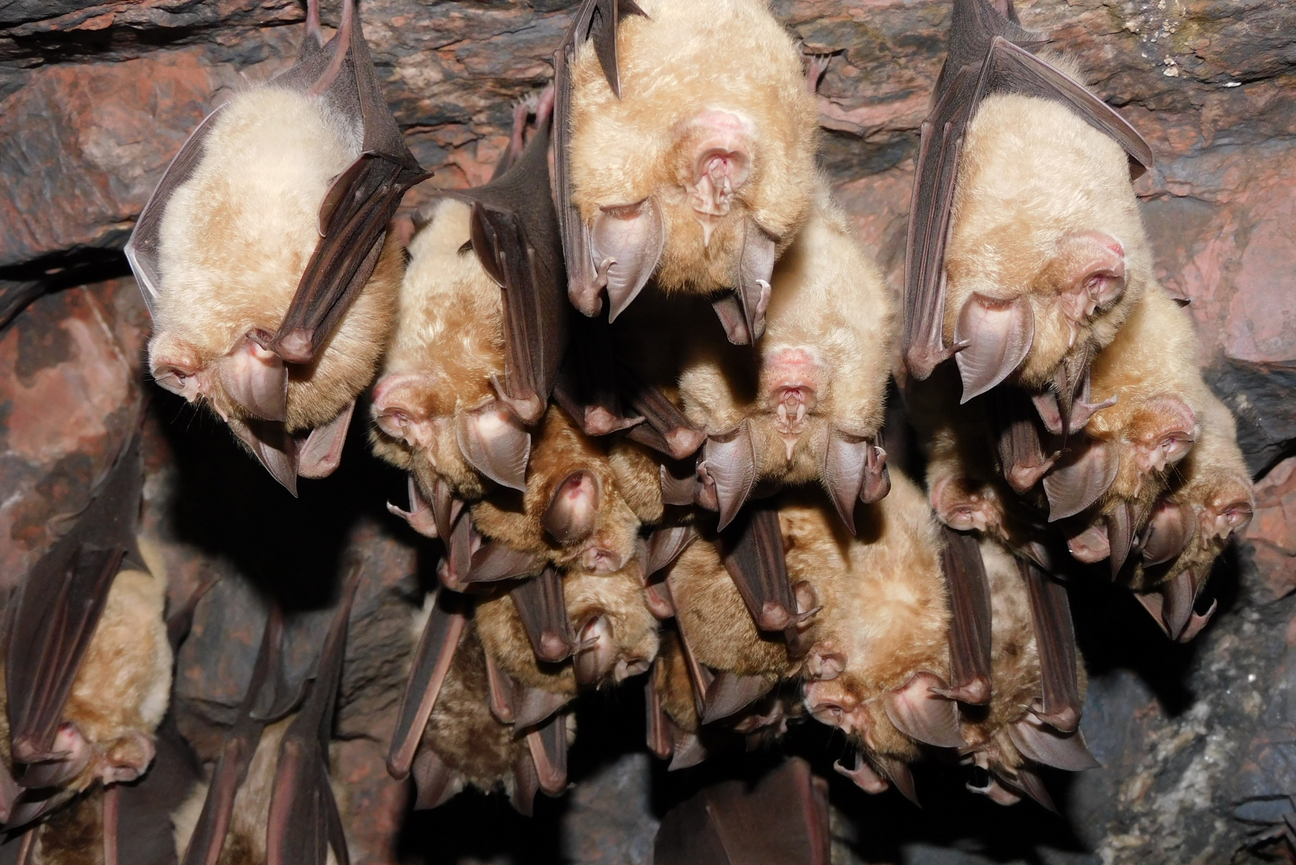
Greater horseshoe bats (Rhinolophus ferrumequinum) are large bats that live in parts of Europe, Asia, and Northern Africa. They use echolocation to hunt their preferred prey, insects. The greater horseshoe bat has an unusual face shape called a noseleaf that helps it to control and amplify the noises it makes in order to improve its echolocation.
Why did Zwaka pick the greater horseshoe bat, specifically, for his stem cell model? One reason is that the species is known to carry a bat coronavirus very similar to SARS-Cov-2, the human COVID-19 virus. This fact also caught the attention of Whitehead Institute Member Rudolf Jaenisch and postdoc in his lab Punam Bisht, who were studying SARS-CoV-2 and other viruses. The Whitehead Institute researchers reached out to Zwaka. Impressed by their stem cell expertise, Zwaka agreed to provide bat cells with which they could generate their own stem cell lines. Soon, Jaenisch and Bisht were ready to begin using bat cells to explore the mechanisms of viral infection and immune defense.
Bats versus the coronavirus
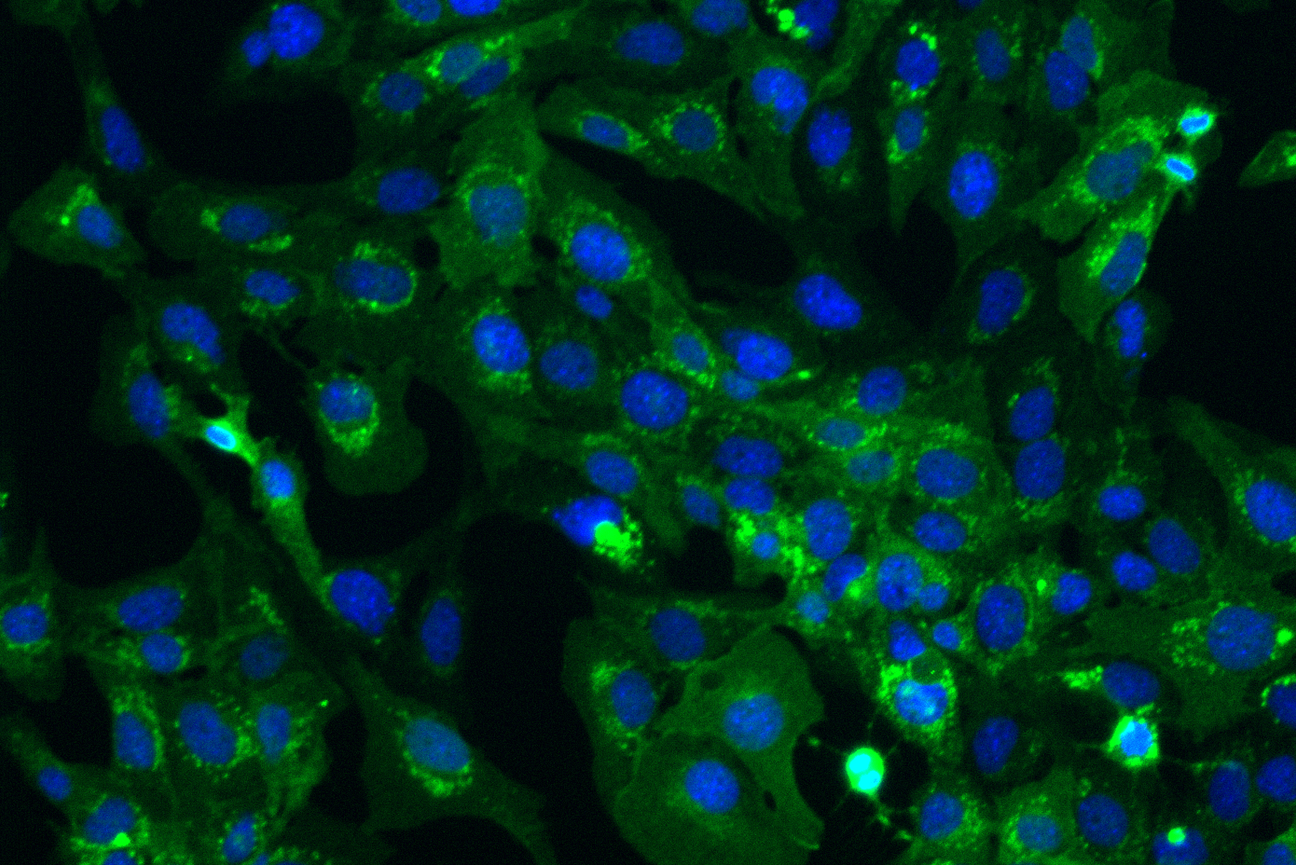
Whitehead Institute postdoc Punam Bisht turned bat stem cells into different cell types that are affected by SARS-CoV-2 (fibroblasts are seen here with nuclei in blue). She also edited bat cells to contain the human ACE2 receptor (seen here in green), which SARS-CoV-2 uses as an entry point to infect cells. Using these bat cells, Bisht has been getting a closer look at how SARS-CoV-2 infects cells and how bat cells prevent the virus from taking over.
Punam Bisht/Whitehead Institute
When COVID-19 first started spreading among humans, researchers tried to identify the source of the virus, and bats were (and remain) a prime suspect. Bats are common carriers of zoonotic viruses, diseases that can “spill over” from one species to another — such as from bat to human, or from bat to another mammal to human. They carry many viruses that are deadly to humans, including rabies, Ebola, Marburg, Nipah, and Hendra viruses. Bats are also known as viral reservoirs: hosts in which viruses can persist and intermittently spread to humans. When humans encroach on bats’ territories — for example, cutting down forests where they live — or when bats’ normal food supply is threatened, then animal and human are more likely to come into close enough proximity for a virus to spread from one to the other.
Unusually tolerant of viruses, bats can get infected and not have serious symptoms. Researchers have studied what makes bats such good hosts for viruses, and what allows them to carry viruses without showing signs of disease. Studies suggest that bats maintain a good balance in their immune response: strong enough to keep the virus from overwhelming their cells, but mild enough not to cause symptoms of disease (most viral disease symptoms are actually caused by the immune system’s efforts to eliminate the virus). When Bisht infected bat cells with SARS-CoV-2, she observed exactly this sort of balance in their response.
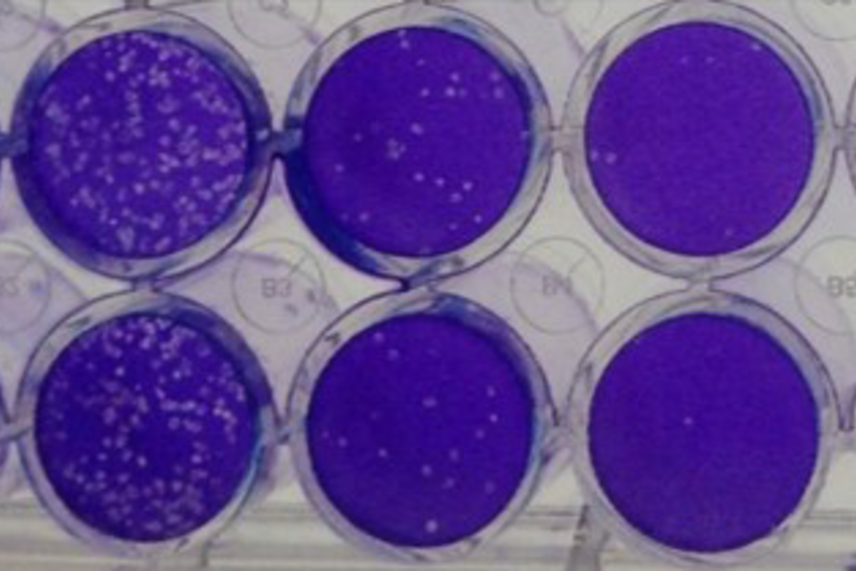
Virus production can be measured in the lab. Bisht can measure how much virus cells are producing using a plaque assay like this one, in which the concentration of viral media is diluted in each dish from left to right and plaques formed by infected cells appear in white.
Punam Bisht/Whitehead Institute
Bisht and Jaenisch published the first of their findings in 2024. They found that bat stem cells had much lower levels of SARS-CoV-2 infection than human stem cells, and that an adult cell type in bats, the connective tissue fibroblast cells, resisted infection altogether. The virus was able to replicate some of its genetic code in bat fibroblast cells, but failed to assemble complete new copies of the virus: this is called an abortive infection. Consistent with previous research, Bisht found that one difference between bat cells and human cells is that the bat cells’ innate immune system is always on high alert, ready to spring into action to subdue viruses immediately, whereas the human innate immune system takes some time to ramp up in response to an infection. This state of constant immune vigilance may be why bat cells are able to quash a SARS-CoV-2 infection before the virus gains control of the cell. At the same time, the bat immune system avoided going into overdrive and creating cytokine storms, a type of immune overreaction that contributed to many COVID-related hospitalizations and deaths.
The researchers observed that the bat cells’ constant vigilance and balanced response is not enough to prevent all viral infections. For example, they found that the Zika virus could mount a successful infection of these cells. They are digging deeper into the genes and pathways involved in order to gain a deeper understanding of how bat cells interact with different viruses.
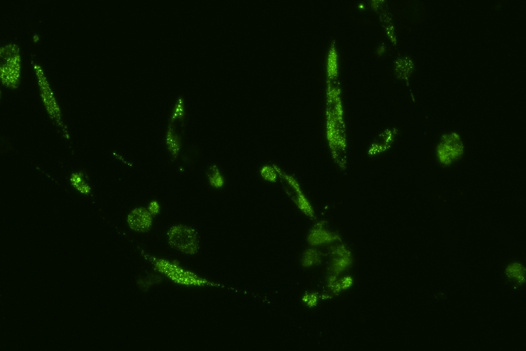
Human cells infected by SARS-Cov-2 (viral RNA in green).
Punam Bisht/Whitehead Institute
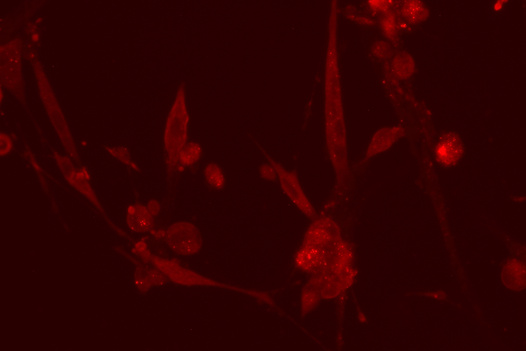
ACE2 receptors, which allow SARS-Cov-2 to enter the cells, marked in red.
Punam Bisht/Whitehead Institute
Bisht’s goal is not only to understand the viruses that we already know about, but also to learn more about zoonotic viruses in general, so that we can be better prepared to protect people against new viruses that arise in the future. Thanks to the development of bat cell lines, Bisht and other researchers have a great new tool with which to conduct this research.
Meanwhile, although bats’ viral tolerance is the trait that first piqued many researchers’ interest in a bat cell line, it is far from the only interesting thing about bat cells. Researchers have been quick to find uses for bat cells in investigations of other biological questions.
Bats run hot and cold
Researchers in Whitehead Institute Member Siniša Hrvatin’s lab study the biology of stasis, periods of low metabolism and body temperature that animals enter to conserve energy. Graduate student Kat Kajderowicz is studying how animals that drop their body temperatures to extreme lows during hibernation survive being so cold. She is using greater horseshoe bats as one species in a comparison of hibernators whose cells can tolerate temperatures down to 4 degrees Celsius. She has observed that the bat cells do remarkably well at such cold temperatures.
Learn more about bat metabolism and energy conservation by using the arrows to go through the slideshow below.
Understanding the biology underlying hibernators’ cold tolerance could help the medical field. Organs and tissues for transplants are kept in cold storage in order to keep them viable. The cold slows metabolism and prevents tissue damage. However, human cells cannot tolerate the cold for long, so doctors have a limited window in which to transport the organ and perform the transplant. If researchers learn how to improve our cells’ cold tolerance, this could keep donor organs viable for longer and reduce the risk of cold damage. The research could also contribute to the Hrvatin lab’s larger goals, which include figuring out whether and how the mechanisms of stasis could be applied to slow down disease and aging in humans.
The fact that a cell line from the greater horseshoe bat proved useful for both Bisht and Kajderowicz’ research is a testament to the intriguing biology of this particular species. However, Rhinolophus ferrumequinum is only one of many bat species, and there is a lot of unusual biology left to be explored across the spectrum of bats.
Getting to know the league of bats
Bats are a diverse lineage containing more than 1,400 species. These different species display a rich variety of traits, providing researchers with lots of biology to explore. Bats come in different sizes: the Kitti’s hog-nosed or “bumblebee” bat is about an inch long with a wingspan of about six inches, while the giant golden-crowned flying fox has a wingspan that can be more than five feet. Bats are found around the world, in all kinds of climates and environments, and these different homes require different traits and behaviors. Some bats roost in trees, others in caves, others in man-made structures. Some species hibernate, some migrate. Some prefer to live alone, while others live in large groups. Bats have diverse diets: some eat fruit or nectar, others eat insects, others are carnivores, and famously, a few species drink blood. Some bats use echolocation to locate their prey, while others rely on eyesight alone.
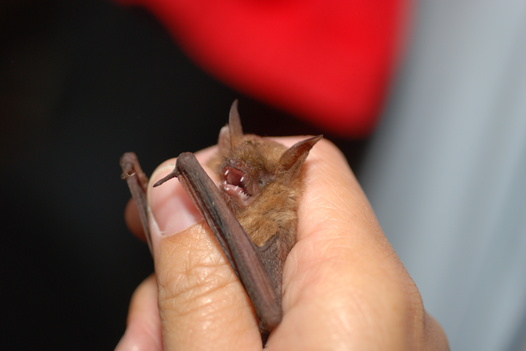
Bats come in all different sizes. The Malayan flying fox (Pteropus vampyrus, right) can weigh roughly 100 times as much as the Geoffroy's bat (Myotis emarginatus, left).
"Capture of Myotis emarginatus" by Gilles San Martin is licensed under CC BY-SA 2.0.
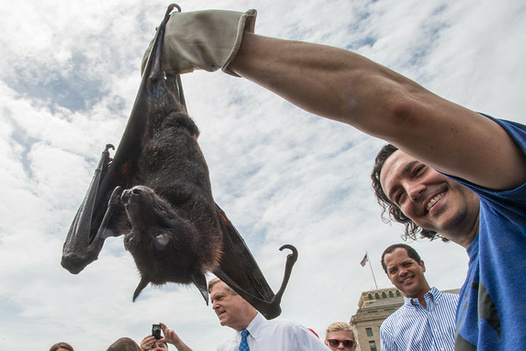
"20150619-OSEC-LSC-0080" by Lance Cheung/USDA.
This wealth of diversity is useful for researchers, because it allows them to make comparisons. If researchers want to figure out the basis for a certain trait, like longevity, they can search for genetic or other biological differences between closely-related bats that either do or do not have the trait. The more similar two species are, the easier it tends to be to spot these differences. Then researchers can test if a difference contributes to the trait of interest.
When Zwaka and his colleagues figured out how to make a stem cell line from greater horseshoe bat cells, they quickly applied the same approach to a second, distantly related bat species, the greater mouse-eared bat (Myotis myotis). Their success suggests that the approach could be applied to any bat species, enabling researchers to generate stem cell lines with which they could investigate unique traits of specific bat species and compare and contrast characteristics across the spectrum of bats.
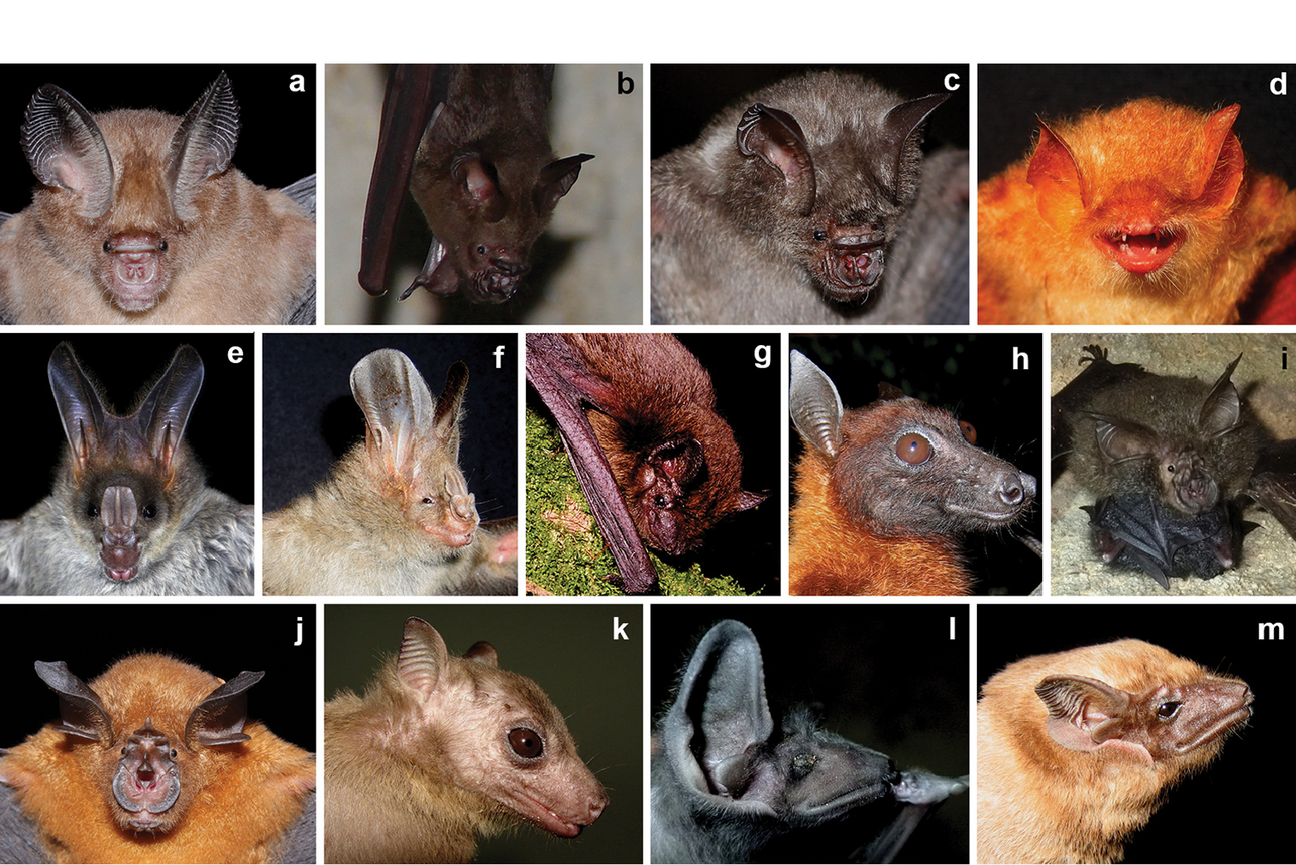
The diversity of bats is exemplified by this collection of bat species recorded around one national park in Sri Lanka. Edirisinghe et al, 2018, figure 2; Bat species recorded in the periphery of Maduru-Oya National Park. a) Hipposideros ater, b) Hipposideros lankadiva, c) Hipposideros speoris, d) Kerivoula picta, e) Megaderma lyra, f) Megaderma spasma, g) Pipistrellus tenuis, h) Pteropus giganteus, i) Rhinolophus beddomei, j) Rhinolophus rouxii, k) Rousettus leschenaulti, l) Taphozous longimanus, m) Taphozous melanopogon.
Figure 2 from Edirisinghe G, Surasinghe T, Gabadage D, Botejue M, Perera K, Madawala M, Weerakoon D, Karunarathna S (2018) Chiropteran diversity in the peripheral areas of the Maduru-Oya National Park in Sri Lanka: insights for conservation and management. ZooKeys 784: 139-162. https://doi.org/10.3897/zookeys.784.25562. Licensed under CC BY 4.0.
Various efforts to collect and make use of more examples of bat biology are already underway – including at a company co-founded by Zwaka, Whitehead Institute Member Richard Young, and National University of Singapore Emeritus Professor and Whitehead Institute alumnus Paul Matsudaira. The company, Paratus Sciences, studies bat biology to develop human therapies.
With projects like this, researchers are only beginning to map the sets of genes and molecular pathways that give bats their incredible resilience. There is a whole world of bat biology as yet uncharted, which researchers now have the tools to explore. The more we learn about bats, the more we can appreciate their unusual biology, and the closer we get to figuring out how we can harness that biology to improve human health.
Contact
Communications and Public Affairs
Phone: 617-452-4630
Email: newsroom@wi.mit.edu