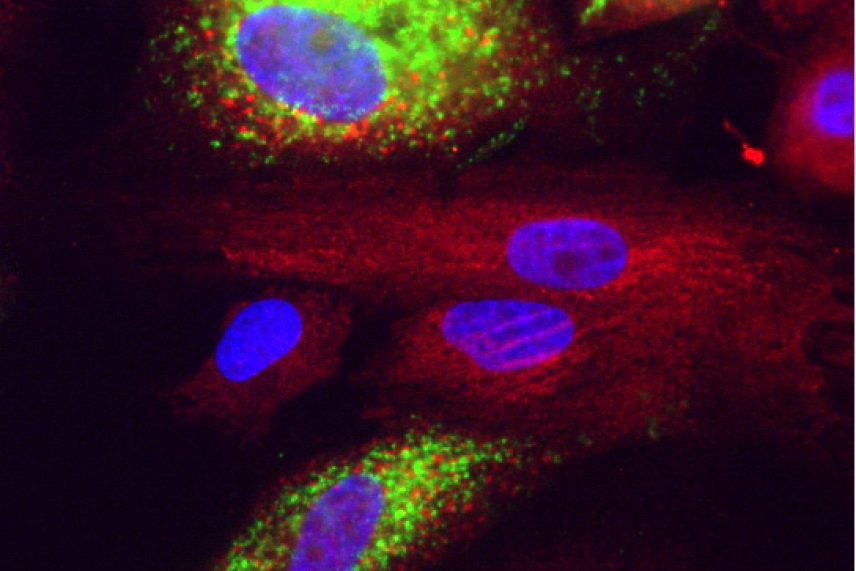
Covid in liver cells. Green is dsRNA, red is calreticulin, blue is nuclei stained with DAPI.
Courtesy of Rudolf Jaenisch
Why RNA research has been critical during the COVID19 pandemic
This story is part of our series, Sculptors of the Cell: RNA research at Whitehead Institute: RNA Research at Whitehead Institute. Click here to see all stories in this collection.
Until the past year, RNA was a molecule mostly relegated to research labs and biology textbooks — an unsung cellular hero. Now, anyone who reads the news can’t help but have it on their mind. The molecule comprises the genetic information of SARS-Cov-2, the virus that causes the COVID19 disease, as well as two of the first vaccines being used to provide immunity against the virus.
Since early in 2020, researchers at Whitehead Institute and around the world have been applying their RNA knowledge to the COVID19 pandemic. In this article, we will explore their research and how advances in RNA biology have helped uncover a light at the end of the pandemic tunnel.
What is an RNA virus, anyway?
The human genome — and that of most other living organisms — is encoded in DNA. But not all living things use DNA as the template for their bodies. “The top 12 deadliest viruses, as classified by the World Health Organization, are all RNA viruses,” says Whitehead Fellow Silvi Rouskin. “That just means that they store their genomes as RNA instead of DNA.”
RNA viruses make up about half of all known viruses. These viruses need to infect hosts with DNA in order to replicate. When an RNA virus like SARS-CoV-2 enters the human body, it attaches itself to the outside of human cells using specialized proteins, and injects the cell with its RNA. The infected cell then treats the RNA like its own, producing proteins from it which are then used to create new viruses. After many viruses have been produced this way, the cell ruptures, unleashing a new generation of viruses to infect other cells.
RNA viruses share some common characteristics beyond their genetic material. For one thing, they usually have a high rate of mutation, meaning that they change often and easily adapt to new conditions. For another, they usually have very small genomes.
“RNA viruses have this challenge that they have to pack so much information in this really small space,” Rouskin says. “So they’ve evolved, and they’ve gotten really creative in optimizing and layering information on top of their primary sequence [the order of the nucleotides that make up the RNA].”
But Rouskin’s previous work on the HIV genome led her to look at other characteristics of the SARS-CoV-2 virus for clues to its function.
“For HIV, there’s only a handful of genes: it actually just has 9 genes — really small,” Rouskin says. “But it needs to express genes from the exact same molecule that is both the genetic material and the thing that gets translated into protein. One way it does this is by folding into certain shapes.”
Using a technique previously developed in her lab, Rouskin and her colleagues were able to discover the secondary structure of SARS-CoV-2’s RNA genome.
Scientists are now studying the different regions of the genome for clues to what the different shapes do throughout the virus’ life cycle, and how this knowledge could be applied to create drugs. “Because those shapes are so important for the function of the molecule, the idea is that by interfering with the shape, then you can cripple the virus in that sense,” Rouskin says. “So it’s a therapeutic-based approach, versus a vaccine.”
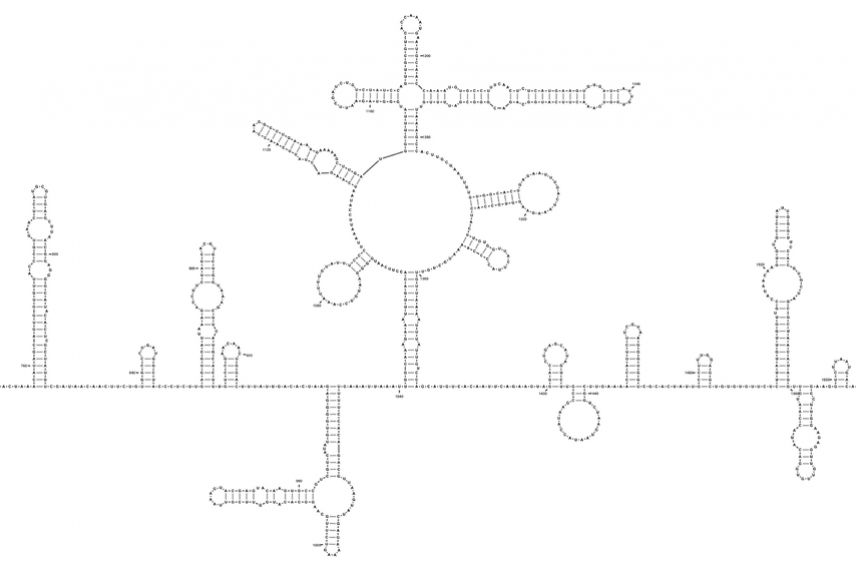
Part of the SARS-CoV-2 genome.
Tammy C. T. Lan et al., bioRxiv
All about RNA vaccines
While Whitehead Institute researchers have been studying possible therapeutic approaches for the virus, other scientists have been tackling the other side of the issue — how to prevent infection by administering a vaccine. As the vaccine rollout reaches more and more people across the country, it’s a good time to reflect on just what a huge step forward these new vaccines are. Traditional vaccines are composed of weakened or inactivated virus proteins. These virus parts act as what are called antigens, provoking the body to develop an immune response without any risk of infection.
The initial vaccines developed for COVID-19 such as those made by Pfizer-BioNTech and Moderna took this method a step further. Instead of providing the body with an antigen, they supply the body with the “recipe” to make the antigen itself in the form of messenger RNA, or mRNA, which is common in the body as an intermediary between DNA and proteins. These two vaccines made history as the world’s first examples of a vaccine approved for human use that used mRNA to confer immunity.
The Pfizer and Moderna vaccines are composed of the messenger RNA that the coronavirus needs to make its spike proteins, proteins on the outside of the virus that help it infect healthy cells. When the mRNA is injected into a person, the person’s cells read the mRNA transcript and create spike protein. Then, the body recognizes the protein as foreign and mounts an immune response, crafting antibodies to find and destroy the spike protein — and whatever it’s attached to. This primes the body for when it encounters a real coronavirus, so it will not have to scramble to throw together an immune response at the last minute.
Drugs that didn’t work — and how we could change that
Throughout the pandemic, there were several examples of drugs that should work against SARS-CoV-2, but for one reason or another, don’t work so well. (One famous example was the broad-spectrum antiviral drug remdesivir).
Whitehead Institute Member Richard Young is investigating how these drugs could be made more effective by building off his past research on small cellular droplets called biomolecular condensates. Condensates bring together the necessary molecules for functions such as transcription, the process of reading DNA into RNA, and previous work from the Young Lab has shown that condensate formation can affect the behavior of drugs in cells.
The Young lab was able to demonstrate in the lab that SARS-CoV-2 proteins form condensates with RNA molecules, and are now working on ways to harness this property to help fight the virus. “We’re figuring out the chemistry that would allow drugs to concentrate in the condensate where [viral RNA] replication is occurring,” Young says.
If their experiments prove fruitful, they could provide another tool in the arsenal scientists have been assembling to tackle SARS-CoV-2 in the future.
Contact
Communications and Public Affairs
Phone: 617-452-4630
Email: newsroom@wi.mit.edu