
Steven Lee/ Whitehead Institute
What can’t plant metabolism make?
This story is part of our series, An energetic pursuit. Click here to see all stories in this collection.
Metabolism is the set of processes that our bodies use to break down and build up molecules as needed for growth and development. It’s how all organisms turn food into the essential building blocks for our cells and energy to keep our cells running. Plants (as well as fungi and bacteria) can perform another set of processes on top of these essential metabolic activities, which enables them to turn the nutrients and energy they absorb into an even greater variety of useful molecules. This additional set of processes is known as secondary metabolism. Over time, plant species around the world have evolved to produce a dazzling array of unique molecules in this way.
Plants generally use the molecules they create through secondary metabolism to respond to changes in their environments. If an animal senses a predator in its environment, it can flee; faced with a long drought, it can migrate. Plants do not have these defenses, so instead they have evolved to become master chemists, concocting unique molecular solutions to their problems. Plants use their specialized metabolic pathways to construct molecules that help them attract pollinators, repel pests and herbivores, protect themselves or their seeds from environmental stressors like UV and drought, and more.
Often these molecules are useful not only to the plants that make them, but also to people. The strength with which plant chemistry can affect humans should come as no surprise to anyone familiar with nicotine, caffeine, or morphine, to name just a few, each of which is a product of plant metabolism. People have been harvesting plants for their unique chemistry for thousands of years. Ancient herbal medicine traditions make use of plants with properties good for pain relief, blood pressure regulation, soothing an upset stomach, preventing or treating infection, and more. Modern science has identified the molecules responsible for many of these plants’ medicinal properties, and plant chemistry has provided the basis for a number of important modern medicines, from the common pain reliever aspirin, to anti-cancer drugs like paclitaxel, to anti-malarial drugs like artemisinin.
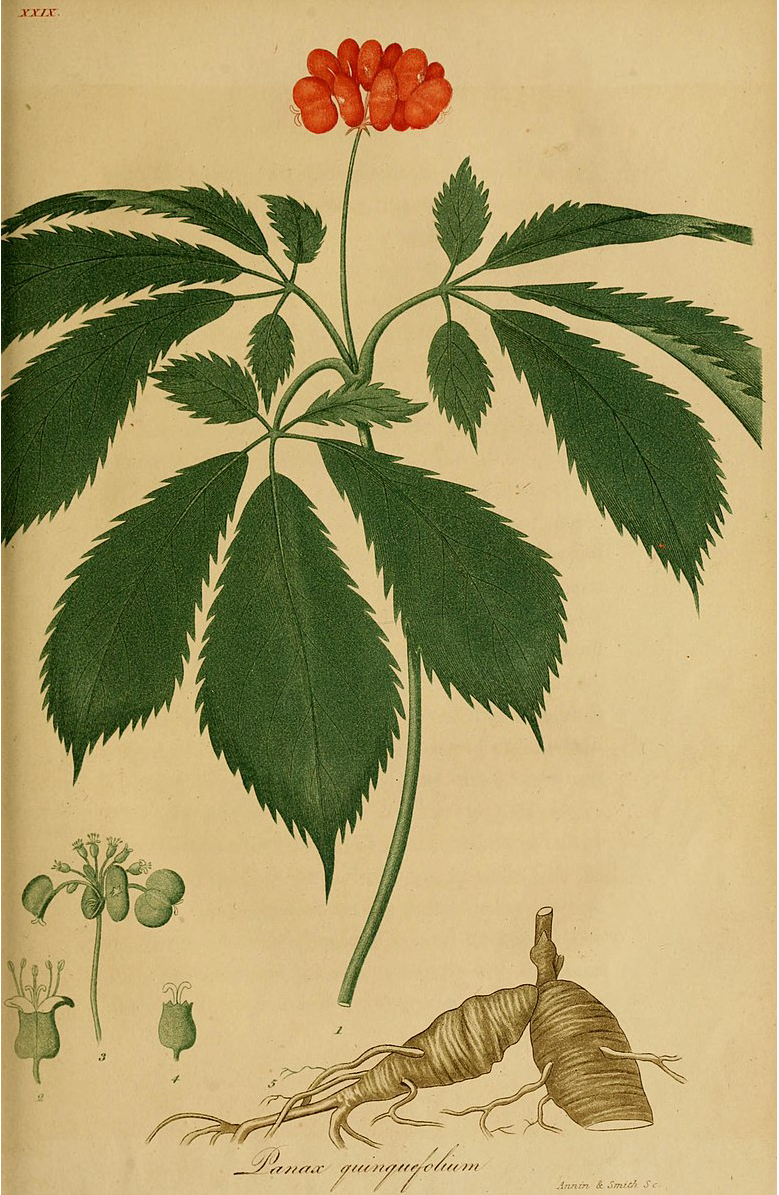
Although numerous molecules of interest have already been discovered in plants, much of the plant world’s chemistry remains uncatalogued. Researchers like Whitehead Institute Member Jing-Ke Weng collect specimens from around the world in search of potent medicines that have yet to be discovered. Weng often turns to well documented herbal medicines of the world as a good starting place for drug discovery, making use of the tradition’s insights into plants’ medicinal properties.
Weng’s lab not only hunts for new plant chemistry, but also seeks to better understand both the molecules of interest that have already been discovered and how plants make these molecules. The metabolic processes that plants use to create molecules of interest can be quite complex, with many steps and genes involved. Understanding these pathways is important because it allows researchers to recreate production of the molecule outside of the plant.
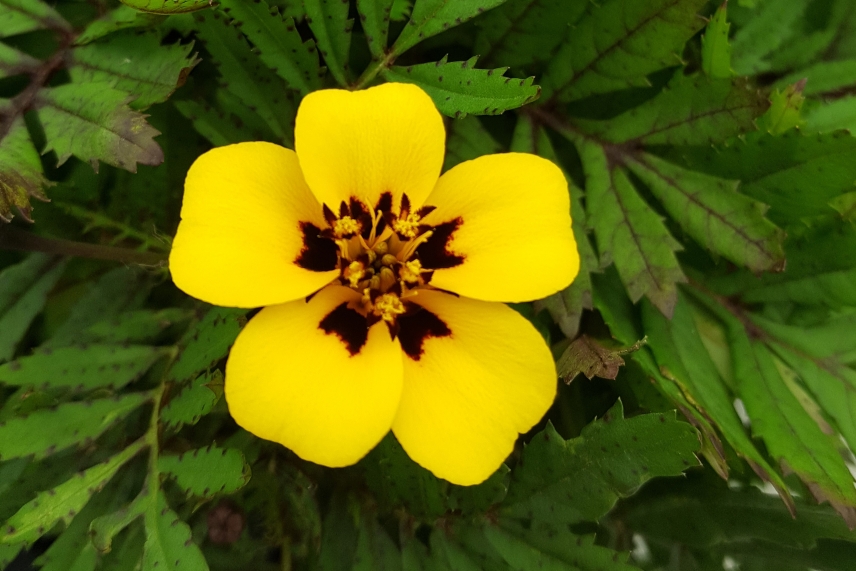
French marigold (Tagetes patula). Weng lab gradate fellow Matthew Hill studies how these marigolds produce thiophene compounds in their roots, which can kill soil nematodes, a crop pest.
Matthew Hill
Historically, useful chemicals have been harvested directly from the plants that make them. Until the recent inventions of certain analytical tools, researchers did not have a good way to figure out how plants were making molecules of interest, and so could not reproduce their production—the molecules are often very complex, and not easy to synthesize from scratch. However, harvesting valuable compounds directly from medicinal plants can be unsustainable. Some plants with useful chemistry are endangered. Some take a very long time to grow and become ready for harvest. If a drug or herbal supplement becomes popular, demand can outstrip what nature can provide. If researchers can figure out how plants make their molecules of interest, then they can recreate that production in a more sustainable system.
Weng’s lab has developed a process to do this. First, they must identify all of the genes that a plant uses to make a molecule of interest. Then, they insert those genes into tobacco, baker’s yeast, or E. coli bacteria, which prompts the new host to begin making the molecule. Each of these alternative hosts is easy to cultivate at scale, in order to mass produce the molecule of interest without any risk of overharvesting. This process for recreating plant chemistry in a new host also enables researchers to modify the production process. They can simply ratchet up the rate of production of the molecule, or they can modify the metabolic pathway in order to tailor the final product. In this way, they can fine tune a molecule of interest from its plant version into a new version that is, for example, a more effective medicine than the original or safer for human consumption.

The tobacco plant Nicotiana benthamiana, one of the common hosts that Weng lab uses to mass produce molecules of interest discovered in other plants.
“Nicotiana benthamiana flower” by Charles Andres, licensed under CC BY-SA 3.0.
In order to identify all of the genes involved in making a molecule of interest, Weng and collaborators must first map out the metabolic pathway that a plant uses to make the molecule. These pathways can be long and complex, involving many steps and genes. Plants’ secondary metabolic pathways are made up of chains of enzymes that function like assembly workers in a factory. The first enzyme takes in a starter molecule and modifies it in some way. Then the next enzyme takes that modified molecule and alters it further. The molecule under construction gets passed through the chain of enzymes, gaining a new modification at each step, until the process is complete and the plant has created the molecule of interest. The process may sound straightforward, but researchers cannot observe it happening, and the genes that code for each enzyme can be scattered throughout the genome, making the configuration of the assembly line a challenging mystery to crack. Researchers must puzzle out the steps using clues from sequencing the genome and transcriptomes of the host plant, tracing metabolites of interest through isotopic labeling, evolutionary biology, and protein structure studies.
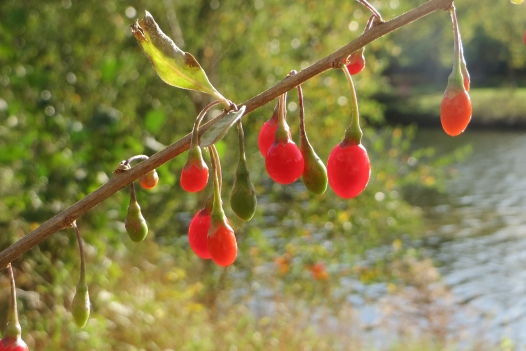
Former Weng lab postdoc Roland Kersten discovered the precursor gene for lyciumins in goji berries (Lycium barbarum).
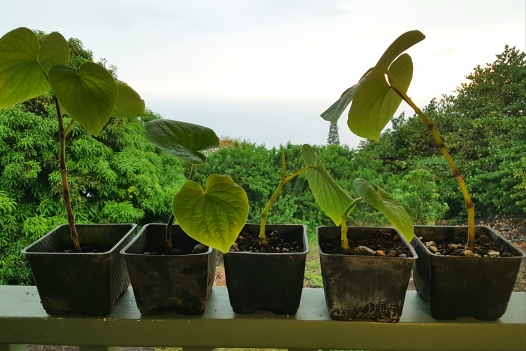
Former Weng lab postdoc Tomáš Pluskal determined how kava (Piper methysticum) produces kavalactones.
Randy Travis
In spite of the challenges, Weng’s lab has solved the metabolic pathways responsible for producing a variety of plant molecules of interest, including some that had been longstanding mysteries in the field. For example, salicylic acid is a key hormone that plants use for a number of important tasks, including growth, development, and immune defense against pathogens. As a medication, salicylic acid is commonly used to treat skin conditions like acne and warts, and it is a key ingredient in the production of aspirin. Researchers have been interested in how plants make salicylic acid for decades, but their understanding of the pathway remained incomplete until Weng and then-postdoc in his lab Michael Torrens-Spence solved the final steps in 2019. Weng and his lab members have also solved the pathways by which different plants produce salidroside, which may be useful for treating diabetes, altitude sickness, and a number of neurological disorders; kavalactones, which may be useful as a non-addictive pain relief and anti-anxiety medication; and lyciumins, which may be useful to treat high blood pressure. They have also solved important sections of other pathways, such as for acutumine, a potential anti-cancer drug.
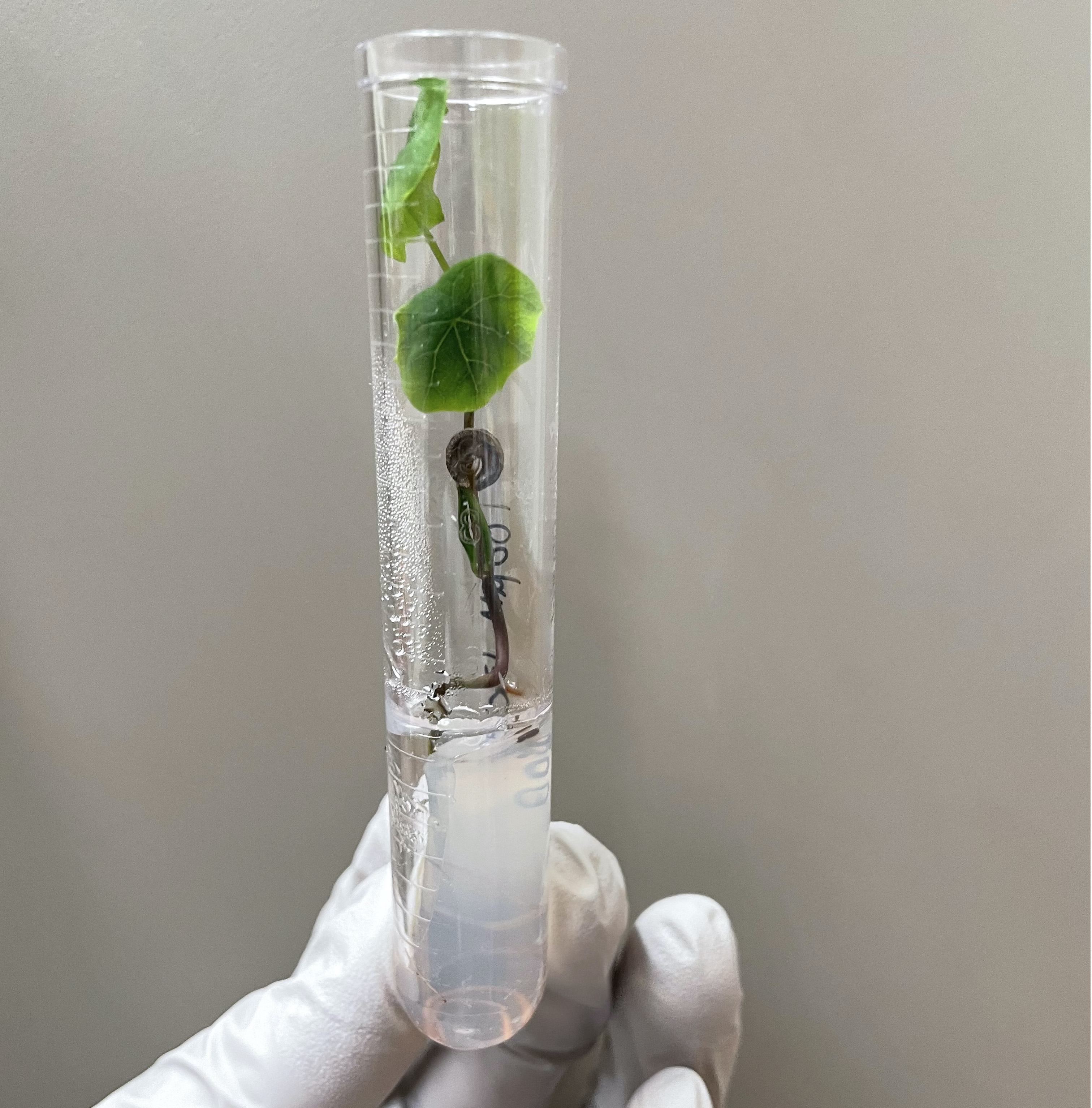
The Weng lab also looks at plants’ metabolic pathways at a zoomed-in level, seeking to understand the structure and function of individual enzymes. At certain points in a plant’s evolution, a copy of an enzyme that it uses to do one thing might evolve to have a slightly different structure. This enables the enzyme to perform a new function, providing the plant with the opportunity to make new kinds of molecules. Understanding how enzymes evolve, and how changes in their structure inform their function, gives the researchers deeper insight into how plants make their molecules of interest. Once researchers understand the role of each enzyme, they can also engineer novel metabolic pathways by stringing together chains of enzymes to produce a desired molecule—something the Weng lab has experimented with.
The tools and processes that the Weng lab has built to understand plant metabolic pathways and then recreate them in new hosts, or alter them to produce tailored molecules of interest, could be incredibly useful for fields including medicine, agriculture, and environmental conservation. With this approach, researchers can sustainably mass produce plant-based medicines and other useful products. They can also engineer plants—for example, by ratcheting up plants’ production of salicylic acid, researchers could make plants more resistant to pests and pathogens, decreasing the need for pesticides. Building on his lab’s previous work, Weng is currently working on engineering plants to sequester carbon more permanently. Plants are already a good carbon storage system, helping to reduce the buildup of greenhouse gases in the atmosphere that contributes to climate change. However, when plants decay, that carbon gets released back into the atmosphere. A few of the molecules that plants make are resistant to decay, such as sporopollenin. The Weng lab determined the detailed molecular structure of pine sporopollenin in 2019, revealing the structural basis for the extreme inertness of this unique biopolymer. Weng wants to see if plants can be engineered to generate lots more sporopollenin, thereby making their carbon storage more permanent. This project is still in its early stages, but such ambitious ideas are only possible because of the knowledge of plant metabolism that Weng and collaborators have developed. Plants have always been a rich resource for humanity, and by gaining a deeper understanding of their metabolic processes, researchers like Weng hope to harness their full potential.
Contact
Communications and Public Affairs
Phone: 617-452-4630
Email: newsroom@wi.mit.edu