Gretchen Ertl/Whitehead Institute
How plants manage difficult decisions
This article is part of our Building a Body story collection. Read the entire collection here.
“Plants are really exquisitely sensitive to the environment,” said Whitehead Institute Member Mary Gehring. “They have to be because they stay in one place. A plant will incorporate information about day length and about patterns of temperature that tell it when to make various transitions — for example when to flower and when to make seeds.”
Gehring’s lab is focused on understanding how plants express the right genes at the right times to have the best chance at survival. By studying a relative of Canola and mustard called Arabidopsis thaliana, Gehring and her lab tease apart the ways plants sense and respond to their surroundings as they are creating and changing their physical forms. These essential changes are implemented through altering how DNA is expressed in the plant.
“I think most people, when they think of DNA, they think of it as fixed, almost like computer code in your cells which is always going to be read the same way,” said former Gehring lab postdoc Ben Williams (now an assistant professor at the University of California, Berkeley). “The problem with that is it obviously has limited flexibility. It might be that you want to read the DNA differently in one cell versus another, and so cells evolved a bunch of different ways to kind of organize their DNA differently.”
The strategies by which cells adjust their gene expression without changing the DNA sequence are called epigenetic. If you think of an organism’s life as a novel, you could think of DNA as providing the dictionary — all the words used to write the story. But if you were to read the dictionary front to back, it wouldn’t have a plot. An organism needs more than just the words to create a full arc of its life cycle; as it moves through the different stages of development, it uses epigenetic marks as one strategy to rearrange the DNA into ‘sentences,’ by expressing different genes at different times. Some genes are used constantly, while others only for a few minutes. Some are used regardless of the environment outside the plant; others are activated based on specific stimuli.
In this installment of our Building a Body collection, learn about epigenetic pathways involved in key steps in a plant’s life cycle, and meet Whitehead researchers studying how plants can act in response to the changing environments around them.
Starting from seed
The life story of a plant begins with a seed. Once a seed has left the parent plant, it is faced with the critical decision of when to germinate. If it germinates too early, the seedling could die in the cold winter temperatures. If it waits too long, it could be crowded out by other plants, or miss its perfect window of temperature and rainfall. Because of these considerations, a seed’s life depends on staying dormant until they encounter the exact right conditions.
Two hormones, abscisic acid (ABA) and gibberellin (GA), preside over the decision of when to germinate. The two hormones are antagonistic — GA promotes germination, while ABA prevents it. The production of each is influenced by outside factors, such as water availability or temperature.
An Arabidopsis seed usually consists of around 3,000 to 4,000 cells. While the seed lacks a central “brain,” the decision of when to germinate has been linked to two small groups of cells clustered in one end of the seed, where ABA and GA hormone production was located. Each clump of cells produces one kind of hormone and sends them to the other clump. When GA becomes the dominant hormone, the clumps of cells send messages to the rest of the seed that it is time to sprout.
One key epigenetic mechanism, called methylation, silences stretches of DNA by tacking chemical groups called methyl groups to individual pairs of “letters” on the DNA strand. This renders the strand unreadable to the cell’s gene expression machinery until the methyl groups are removed. As the process of germination progresses, the seed undergoes widespread demethylation, and many genes that were silenced in the dry seed are then activated as the seed comes out of its dormant period. This demethylation likely happens passively, without the help of specific demethylation enzymes (more on these later).
Finding the time to flower
The next big decision for many plants is when to make their flowers. “Flowering time is dependent on temperature, pests, when the pollinators are around — and the timing of that is super important,” said Williams.
Williams led a study published early this year that uncovered the role of one particular class of genes in regulating this important decision. In Arabidopsis, the DEMETER gene family plays a role in regulating methylation. These gene’s protein products, called demethylases, pluck off methyl groups from already methylated and silenced regions of the DNA strand, allowing genes on those parts of the strand to be expressed in the cell.
In the past, researchers had struggled to identify the specific contribution of one DEMETER gene, called DME, because DME is essential for proper seed development. In other words, if they disrupted the gene, plants never grew at all. Williams and collaborators designed an experimental system that allowed DME to be turned on for a brief period of time when it is necessary for reproductive development, and then turned off so scientists could observe what happened to a plant in its absence. “It turns out that DME, one of these DEMETER genes, is really important for flowering time,” Williams said.

Arabidopsis thaliana
"Arabidopsis thaliana 2019-04-27 2246" by Salicyna is licensed under CC BY-SA 4.0.
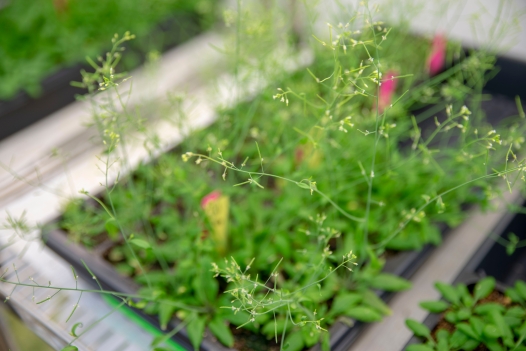
Flowers of the model plant Arabidopsis thaliana.
Conor Gearin/Whitehead Institute
Plants with DME knocked out did not heed environmental cues about when to flower — they just did it. “It was almost like they weren’t putting any effort into the decision,” said Williams.
This discovery helped Williams refine his big-picture view of how plants use epigenetics in decision-making. “When I zoom out, I think about how one of the benefits to epigenetics as opposed to genetics is it could give a plant more flexibility in the timing of decisions,” he said. “If you have to make a decision in a timescale of several months, you can't really evolve like a biochemical pathway that takes months to complete. You need some sort of more stable regulation mechanism. And epigenetics is, I think, a perfect example of that.”
Beginning again
When a flower is pollinated and fertilized seed production can begin, a whole new set of decisions become important. These include how certain parental genes are distributed throughout the seed.
A complete seed is made up of three genetically distinct parts: the seed coat, the embryo, and the triploid endosperm. The seed coat is provided by the mother and protects the seed. Inside are two products of fertilization that come from both parents: the embryo, which will become the new plant, and the endosperm, which plays a similar role to the human placenta, providing the growing embryo with nutrients.
Over the past few years, Gehring and her lab have been studying how parent plant’s genes are expressed in the endosperm. This tissue, with its important role in providing “food” for the growing embryo, is the site of genetic conflict between mother and father plants. Because mother plants can be fertilized by a number of fathers, there’s a built-in competing interest between the two parents. It’s in the interest of each father to hog as many nutrients for their specific offspring as possible, while the mother benefits from as many of her seeds from different fathers getting enough nutrients to survive to adulthood.
The outcome of this conflict plays out through an epigenetic mechanism called imprinting. Through targeted methylation, either the mother’s or father’s copy of a gene is silenced so that only the other parent’s copy is expressed in the offspring. If the fathers’ genes win out, the seeds are generally larger. In some cases, when the ratio of paternal to maternal genes was off, seeds aborted before reaching maturity.
Gehring and postdoctoral researcher Satyaki Rajavasireddy recently discovered that both parent plants use a specific enzyme, RNA polymerase IV, as a tool to influence gene expression. But while RNA polymerase IV is used to further the conflict between parent plants, Gehring hypothesized that it could also provide a way to reach a consensus that was acceptable to both parents.
Harnessing epigenetics
Gehring’s work to understand epigenetic mechanisms behind plant decisions has real-world applications. Working in collaboration with Jing-Ke Weng and Jonathan Weissman, Gehring is contributing her expertise to an ambitious climate change project with the aim of creating hardier crops. Gehring is focusing on a phenomenon that occurs when two varieties of plants are crossed — the immediate offspring are often healthier and more vigorous than the previous generations. This advantage, however, is often lost in subsequent generations.
“We'd like to use our knowledge of the genetics and epigenetics of seed development to be able to maintain that vigor by making asexual seeds that are actually clones of that hybrid vigorous plant,” she said. “In this way the progeny of that hybrid plant will have the same phenotype and the same traits as the parent.”
Her lab has been working to initiate seed development without DNA from sperm. “If we're successful this approach will both hasten breeding programs, and it'll give us this ability to maintain desirable traits from one generation to the next,” she said.
She’s also studying the potential of orphan crops, or crops which have significant regional importance but are not traded globally. “We want to take what we've learned from epigenetic engineering in Arabidopsis seeds, and then take that into some of these orphan crops to see whether or not we can manipulate epigenetic markers to turn genes on and off at certain times during seed development to alter seed characteristics,” she said.
One crop she’s started to work on is a legume called the pigeon pea, which is a major source of protein across South Asia, Africa and the Caribbean. “We're really excited to actually start taking what we've learned from model species and seeing if we can apply these lessons to crops that may be more successful in our changing planet,” she said.
Contact
Communications and Public Affairs
Phone: 617-452-4630
Email: newsroom@wi.mit.edu