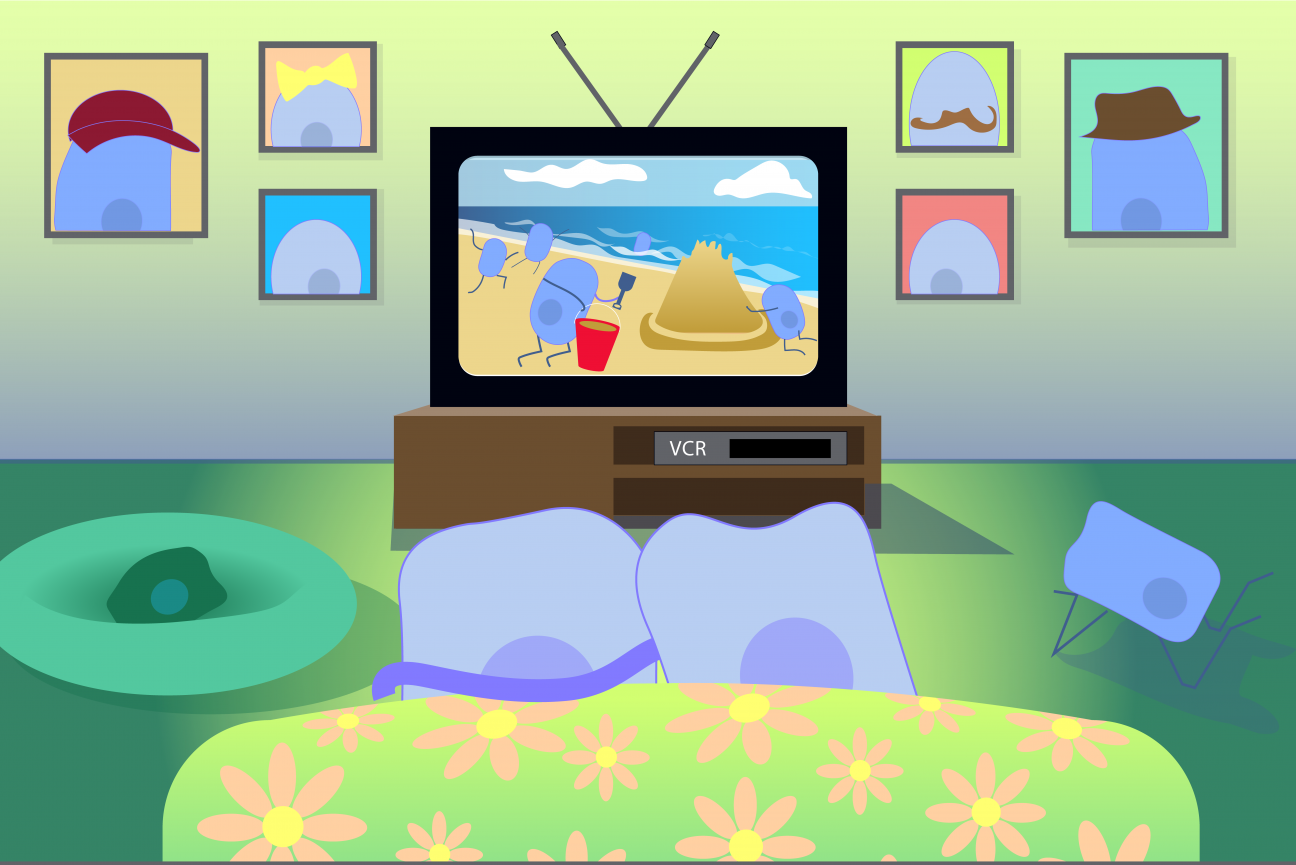
New technologies in the field of cell tracking are allowing researchers to see and continuously record cellular events as they happen in real time — more like a home video than a static portrait.
Jenn Cook-Chrysos/Whitehead Institute
Catching key moments of cancer progression
This story is part of our series, Cells Over Time. Click here to see all stories in this collection.
Important moments of cancer — mutation, tumor formation, metastasis — are fleeting, easy-to-miss events. Even with modern medical technologies and methods, they often happen unobserved, and are only realized later when these cells spawn life-threatening conditions.
In recent years, however, new methods of tracking individual cells through time have allowed researchers to get closer to the origin of these events, and Whitehead Institute scientists are turning the power of these technologies to study cells involved in several different types of cancer. “With [these technologies], you can track down rare events in the past, identify all the offspring of an event, and see how they've changed their behavior,” said Whitehead Institute Member Jonathan Weissman. “You can ask, when a cell picks up an oncogene, how does it mutate, and further evolve, and proliferate and metastasize?”
Read on to learn how three Whitehead Institute Members are using specially engineered mice, CRISPR-based technologies, and other methods to track cells at different stages of cancer development, pushing the boundaries of what we understand about how the disease starts and proliferates. From the initial beginnings of a tumor, sometimes in the darkness of a still-forming embryo, to a tumor’s growth and eventual metastasis to other sites in the body, Whitehead Institute scientists Rudolf Jaenisch, Jonathan Weissman, and Robert Weinberg study the pivotal points in a cancer’s growth and spread.
The birth of a tumor
For around 800 children each year in the United States, the seeds are sown during fetal development for a rare and unpredictable childhood cancer called neuroblastoma. To understand how the disease develops, scientists need to study what happens to these cancerous “seeds” as the embryo matures. But they can hardly study a living human embryo, and fetal development is such a complex process that it is near impossible to simulate it in the lab.
To make matters more complex, the cancer grows and then may shrink unpredictably after the children are born and as they age. Sometimes the tumors disappear on their own; other times they grow uncontrollably.
In 2020, Institute Founding Member Rudolf Jaenisch’s lab introduced a new way of tracking the cells involved in the disease using his tried and true method for modeling such complex conditions: chimeric mice. A chimera is a conglomeration of two species — in this case, mostly mouse, with a few strategically placed human stem cells.
To create chimeras to study neuroblastoma, Jaenisch, who is also a Professor of Biology at Massachusetts Institute of Technology (MIT), along with collaborators at the Koch Institute for integrative Cancer Research at MIT and the Dana-Farber Cancer Institute, engineered human stem cells with glowing proteins to make them easy to see under the microscope. The cells contained a special genetic-switch that allowed the researchers to induce tumors by adding a certain chemical. These human stem cells were then induced to form a more specialized cell type – a neural crest stem cell.
Neural crest cells are a group of developing stem cells that go on to form the peripheral nervous system as well as other parts of the body such as the facial bones. It is neural crest cells that mutate into neuroblastoma tumors in humans, so the researchers hoped that by using these cells, they could create “human” tumors in mice. The researchers injected these human neural crest cells into mice so that they could readily incorporate with the host’s cells.
After the mice were born, the researchers were then able to take samples from the mice over the course of their development to see whether these implanted cells would form neural crest-derived tumors and, if so, what happened as they grew — something they would never have been able to do with neuroblastoma tumors in human infants and children.
The tumors in the chimeric mice pups developed in similar forms to human neuroblastomas — specifically, they formed characteristic rosette shapes — very similar to those seen in patient’s samples. With the help of Stefani Spranger, an assistant professor of biology at MIT and a Member of the Koch Institute for Integrative Cancer Research at MIT, they were able to track the cells’ interactions with the mice’s immune systems, and learn how the cancer “tricks” the immune cells into letting it stick around.
Now that they are able to model the formation of neuroblastoma tumors, the researchers hope to find a way to eliminate the tumors in the mice. “This is a model that will allow us to approach how to get rid of the tumors,” said Malkiel Cohen, a former postdoc in Jaenisch’s lab and first author of the paper, published in the journal Cell Stem Cell describing the work.
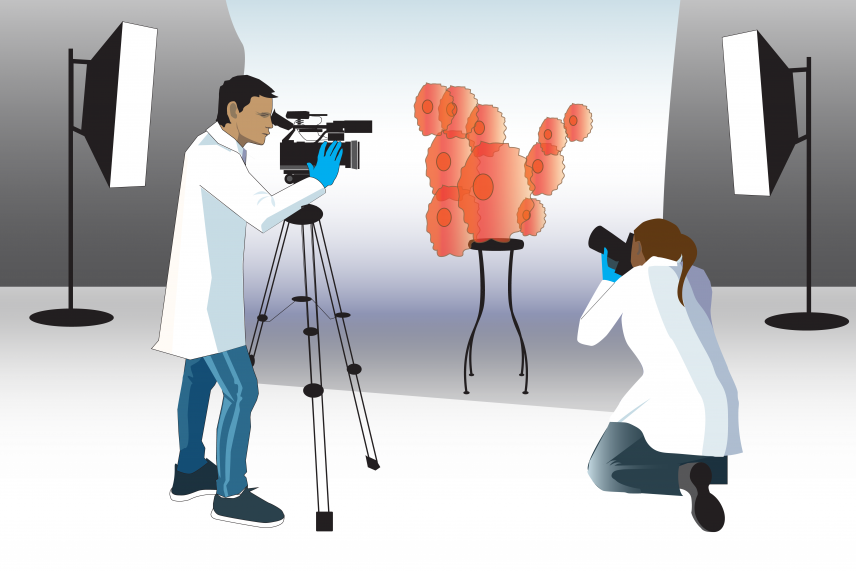
Jennifer Cook-Chrysos/Whitehead Institute
Cancer genealogy
A recent project from the lab of Whitehead Institute Member Jonathan Weissman focuses on another essential moment of cancer progression: metastasis. Metastasis happens when cancer spreads from a primary tumor to distant places in the body. Weissman, an expert in genome editing, created a CRISPR-based method to track the lineage of individual cancer cells in real time as they proliferate and metastasize.
Weissman and his collaborators, including graduate student Matthew Jones and then postdoctoral researcher Jeffrey Quinn, adapted the technology from a similar tool designed by Michelle Chan, a former postdoc in Weissman’s former lab at the University of California, San Francisco (UCSF) who is now an assistant professor at Princeton University. Chan designed a CRISPR mechanism to track the lineages of embryonic cells as they developed into specialized tissues.
“What Michelle Chan was able to do was uncover how tissues that look very similar to one another, actually come from disparate sources,” said Matthew Jones, a graduate student in Weissman’s lab and a co-first author on the paper describing the new method. “That has rapid implications about how tissues organize themselves, and we wanted to apply it to cancer.”
To create this system, the researchers engineered cancer cells with added genes: one for Cas9, the gene that codes for CRISPR’s “molecular scissors,” others for fluorescing proteins for microscopy, and a few sequences that would serve as targets for the CRISPR technology.
They then implanted thousands of these cells into the lungs of mice to simulate a tumor, using a model designed by Trever Bivona, a cancer biologist at UCSF. As the cells in the model tumors began to divide, the Cas9 protein began making small snips in the target sites in the cells’ DNA. When the cells fixed these snips, they patched in or deleted a few random nucleotides, leading to a unique “barcode” in each cell. Because these barcodes were added to each cell’s DNA, they were heritable and able to be passed on through generations of cells.
With the help of Nir Yosef, a computer scientist at the University of California, Berkeley, the researchers organized the data into “family trees” of cancer cells spanning multiple generations. By taking samples from different areas of the body, the researchers were able to resolve exactly when a cell jumped from where it started, in the lungs, to a distant tissue.
When they compared the trees, the researchers noticed that some cells were highly metastatic, jumping around multiple times over the course of the experiment, while others stayed put throughout. “We were excited to uncover some of these really rare, but consequential events that happened in the past that you would never be able to observe, and rarely be able to infer from a static snapshot,” Jones said.
By comparing highly metastatic and non-metastatic cells, they were able to identify metastasis-associated genes and answer questions about how the tumors were evolving and adapting. “It’s an entirely new way to look at the behavior and evolution of a tumor,” Weissman said. “We think it can be applied to many different problems in cancer biology.”
The next steps
A natural tumor begins with a single cell, mutated in a way that leads it to “go rogue,” so to speak. To mimic this in a model system, Dian Yang, another post-doc in the Weissman Lab, is collaborating with researchers in the lab of MIT Professor of Biology and Koch Institute Director Tyler Jacks’ to create a mouse model with the CRISPR lineage recording tool embedded in its DNA.
The model is based on a mouse model created by Jacks’ lab to study lung cancer. The lab has created genetically engineered mice that when left alone, live completely normal lives. But upon adding a trigger (an enzyme called “Cre”) — in Jacks’ and Yang’s case, they deliver the trigger to the lung using a virus — oncogenic signals are activated and lead to spontaneous tumors in the mice’s lungs.
Being able to “switch on” the mouse model in this way has a number of advantages. “Each tumor will start from one single transformed cell, which we can then watch in its native environment as it evolves,” Yang said. “Then, we can look back later at how the cells metastasize.”
Adding the CRISPR system to Jacks’ existing cancer model will also allow researchers to study cancerous cells on a broader time scale. “Usually, when we harvest samples from the mice, it is like a snapshot, just one sample at one stage,” Yang said. “You can see what it looks like, you can analyze gene expression at the time of sampling, you can even take a time series, but you don't know what happened in the past.”
In a sense, the lineage recording technology embedded in the genome of the mice now makes it possible to look back in time. “When you have a million cells in a tumor, you can use the lineage network of these cells to find out how they're related, and connect the current state with the past evolutionary lineage history,” he said. “I think this will provide a new dimension of biological information for us to understand biology that is not just limited to cancer biology.”
The making of metastasis
Weissman’s lab’s method for tracking the lineage of cancer cells can illuminate the nature of the cells that leave the primary tumor and scatter throughout the body. But what actually happens to these cells to cause them to metastasize?
That’s where Whitehead Institute Founding Member Robert Weinberg’s research comes in. Weinberg has been studying cancer for decades. His early work helped to answer the question of how cells that form a primary tumor become cancerous. Weinberg identified the first human oncogene, a gene that causes otherwise-normal cells to form tumors. This finding, combined with others, demonstrated that cancer is a disease driven by damaged genes, at least in its origins. Weinberg has since turned his attention to the question of how cancer cells acquire the ability to spread.
Around 90 percent of cancer deaths are caused not by the primary tumor, but by its metastasis. Based on previous work, there is no single genetic switch that can be flipped to equip a cancer cell for metastasis. Instead, cells must go through a series of changes over time. Most cancer cells fail to acquire all of the necessary traits, and so may, for example, spread to new tissues but rarely form tumors there. Weinberg’s lab tracks cancer cells to help fill in the “road map” that cancer cells follow on the way to metastasis, in the hopes that their insights can be used to prevent or treat metastatic cancers.
One important change that cancer cells undergo is called the epithelial to mesenchymal transition (EMT), a cellular process that causes the cancer cells to express different genes and go from being immobile to mobile and invasive. Cancer cells undergoing this transition to be able to spread are called “quasi-mesenchymal.” The ability to spread does not fully explain metastasis, however.
“There's two aspects of the metastasis problem,” Weinberg said. “The first aspect is how cancer cells physically leave the primary tumor and get seeded into a distant tissue. In other words, the physical translocation of the cells. The second step represents the subsequent ability of the already seeded cells to figure out how to make a living in a distant tissue.”
In other words, how do those transplanted cells adapt to a new tissue environment, which might offer them only inhospitable conditions? “That represents the major unsolved problem of metastasis,” he said.
Weinberg hopes to study this more in the future; for now though, his lab has found that studying quasi-mesenchymal cells can serve another purpose. Anushka Dongre, a postdoc in Weinberg’s lab, found that these cells are resistant to a common type of cancer treatment, known as immune checkpoint therapy, and can even protect the other cancer cells around them from that therapy. If as little as ten percent of a tumor consists of quasi-mesenchymal cells, then the whole tumor may become resistant.
By using a tumor’s epithelial/mesenchymal profile, Dongre demonstrated that she could predict how likely a breast cancer tumor was to respond to a particular immune checkpoint therapy. This finding could help physicians match patients to the best treatment plan, by indicating ahead of time whether the treatment will work. She also identified a way to eliminate the quasi-mesenchymal cells’ protective effect by suppressing a certain enzyme that they employ to defend themselves.
Weinberg’s lab continues to study pivotal changes in the lives of cancer cells, such as the EMT, so that they can better understand metastasis and, they hope, help find effective treatments for patients with metastatic cancers.
Tracking cells into the future
Scientists have been tracking cells for more than a century, and Whitehead Institute scientists will be tracking cancer cells for decades to come. In the coming years, Weinberg plans to continue to investigate the mysteries of metastasis. For Weissman’s part, he hopes to continue refining his CRISPR technique, with the end goal of eventually being able to predict the behavior of cancer cells. “We want to be able to measure where they are, where they're going at any time, and then predict where they're going to be in the future,” he said.
With new technologies and ever-expanding fields, there is limitless potential in these various methods. “That’s what is so exciting about the cell tracking field right now,” said Matt Jones. “It's really pushing the boundaries on what we can capture from our measurements.”
Cohen, M., et al. Formation of Human Neuroblastoma in Mouse-Human Neural Crest Chimeras. Cell Stem Cell. March 5, 2020. DOI: https://doi.org/10.1016/j.stem.2020.02.001
Dongre, A., Rashidian, M., Eaton, E. N., Reinhardt, F., Thiru, P., Zagorulya, M., Nepal, S., Banaz, T., Martner, A., Spranger, S., & Weinberg, R. A. (2020). “Direct and Indirect Regulators of Epithelial-Mesenchymal Transition (EMT)-mediated Immunosuppression in Breast Carcinomas.” Cancer Discovery, online Dec 16, 2020. DOI: 10.1158/2159-8290.CD-20-0603
Jeffrey J. Quinn, Matthew G. Jones, Ross A. Okimoto, Shigeki Nanjo, Michelle M. Chan, Nir Yosef, Trever G. Bivona, Jonathan S. Weissman. “Single-cell lineages reveal the rates, routes, and drivers of metastasis in cancer xenografts.” Science, Jan. 21, 2021.
Contact
Communications and Public Affairs
Phone: 617-452-4630
Email: newsroom@wi.mit.edu