Array for the cell
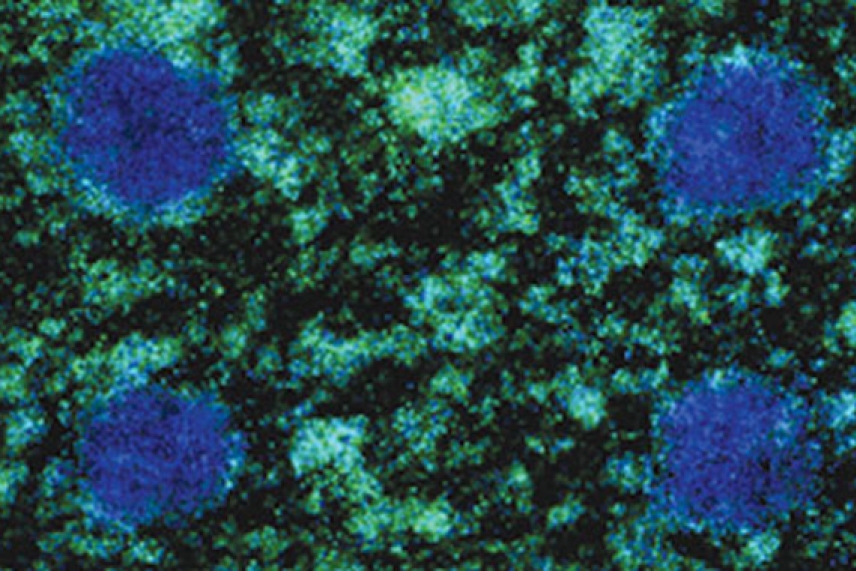
Closeup of an array, with spots that are alive (blue) in a field of dead cells.
Image: Douglas Wheeler
Figuring what a gene does is hard work, but it’s vastly easier than it was a few years ago. Back then, you would laboriously isolate a single gene, tinker with it to get some inkling about its purpose, and then start speculating about how it might collaborate with other genes. Now, microarrays let researchers gather exponentially more data about gene expression––what each of thousands of genes does, perhaps in coordination with other genes.
The microarray was born a decade ago as a solution to the one-gene-at-a-time dilemma. While studying the growth and development of Arabidopsis thaliana, the mustard plant, Stanford University scientists created a robotic system for screening thousands of genes at once on small glass slides.
By fixing tiny dots or “probes” (each a short bit of DNA or RNA) onto a slide in an organized grid, scientists fashioned a microarray, also known as a gene chip or DNA chip. The dots were so tiny that a slide could hold thousands of probes.
Next, a sample solution of “target” RNA (actually a solution of DNA and RNA from broken cells) was dropped on top of the microarray slide and held by a slide coverslip. Each probe now served as an individual test. When an RNA target came in contact with matching DNA on the slide, it stuck. The target was labeled with a fluorescent tag. The better the match, the more it glowed. Researchers could measure each reaction and discover which genes were active for a given target.
Just a year later, Affymetrix introduced the first commercial microarray. In 1997, the first complete eukaryotic genome on a microarray (baker’s yeast) was published in Science. DNA microarrays vaulted to the forefront of biotechnology, in turn spawning RNA-based arrays and protein-based arrays.
Douglas Wheeler and David Sabatini are developing microarrays for tests with living cells.
|
Researchers in Whitehead Associate Member David Sabatini’s lab now are upping the ante with new cell-based microarrays that promise much for the field of drug research. Unlike traditional cell assays, these experiments can test thousands of living cells at once to study cell pathways and drug interactions, and let researchers examine how these pathways and drug interactions affect each other.
This cell-based microarray technology “has great potential for high-throughput screening,” says Norbert Perrimon, a Harvard Medical School investigator who studies gene functions on a genome-wide scale in the Drosophila fruit fly.
A genome on four slides
The miniaturized aspect of microarrays is key to their allure. Cell-based microarrays will let the Sabatini lab test every gene in the entire fruit fly genome using just four glass slides and a relatively newly discovered phenomenon known as RNA interference (RNAi). The lab recently proved this in principle and is now working its way through the genome.
To use RNAi, scientists synthesize small RNA molecules to target and “knock down” a specific gene by blocking its ability to create protein. Each RNAi molecule is specifically designed for its target gene, so a 20,000-gene genome, such as the fruit fly’s, requires 20,000 RNAi molecules.
While these molecules can be bought or generated in the lab, the sheer volume required is a challenge. The Sabatini lab needs significantly fewer with its microarray format than it did with microtiter plates, a previous technology. On a microarray, each spot needs only enough RNAi molecules to “infect” about 300 cells. In contrast, a microtiter plate well holds many thousands of cells and needs a corresponding number of RNAi molecules. Also, microtiter plates hold 384 wells each. But microarray slides can hold thousands of samples––5,000 in this case.
Cells are then “seeded” on the array. A solution of cells and cell growth media is poured over the microarray slide in a Petri dish. After an hour or two, the cells land on the bottom of the dish and attach. A layer of cells grows over both slide and Petri dish. Cells that land on an RNA spot probe are affected by the probe and their reactions can be analyzed.
Plenty of groups have done RNAi arrays, notes Douglas Wheeler, a technical assistant in the Sabatini lab, but they’ve used mammalian cells. Those have serious technical drawbacks, including a response to interferon proteins (which cells produce in reaction to viral infections) that makes cell uptake of the large RNAi molecules difficult.
Recognizing this, the Sabatini lab committed to the same work in Drosophila. Much of the genome is conserved between fruit flies and mammals, and the fly cells easily take up RNAi molecules. “Because Drosophila is so easy, why not do the initial throwing out of the net with a screen in Drosophila, and then do the follow-up in mammalian cells?” asks Wheeler.
This approach should allow scientists to pin down pathways for cellular growth, with implications for cancer research. “We could knock down every gene in the genome to find which genes affect how fast cells replicate and how big they get,” says research assistant Steve Bailey.
Faster data, faster drugs
In a second line of research, cell-based microarrays let researchers test chemical compounds on cells by the thousand. The more of these “small molecule” compounds tested, the better the chance of finding one worthy of further testing for therapeutic value. Cell-based microarrays, which connect live cells with possible drugs, can churn through large numbers of compounds.
In one such experiment, Bailey used a robot to print the same 72 compounds onto a series of slides. To hold the compounds in place, he embedded them in a biodegradable polymer, creating a series of tiny parallel dots. “They actually look, at the microscopic level, like Braille,” says Bailey.
Bailey then covered the slides with cells. The polymer slowly dissolved, and he measured how the cells reacted to the compounds. This technique, he suggests, could be applied to cells that mimic cancer cells. If you combine these cells with myriad compounds, and one compound in particular kills the cells, you might just have a potential drug to fight cancer. The lab hopes to license the technology.
The microarrays can do “double knockdowns,” manipulating more than one gene at a time to see if and how they’re connected. “One can very quickly go through very large numbers of genes and very large numbers of cell phenotypes and say what genes are responsible,” says Sabatini.
“These microarrays allow use of rare cell types (because we only need a few cells per experiment), allow use of rare compounds, and allow imaging of cellular functions and molecules,” adds Brent Stockwell, an assistant professor at Columbia University and former Whitehead Fellow who developed the microarrays jointly with Sabatini. “They should accelerate both basic biology and drug discovery efforts.”
Jennifer Tomase is a writer at Harvard University. This article first appeared in the Spring 2005 issue of Paradigm magazine.
Topics
Contact
Communications and Public Affairs
Phone: 617-452-4630
Email: newsroom@wi.mit.edu