Unusual Labmates: Nature’s Peter Pans
Axolotls (Ambystoma mexicanum) are a critically endangered species of salamander. The species has only one natural habitat remaining, a series of canals in Mexico City, and only 50-1000 axolotls are estimated to be left living there.[1] However, axolotls have become popular both as pets and as model organisms in research, so descendants of axolotls collected from Mexico City many years ago can now be found around the world. Axolotls are highly regenerative, able to regrow entire limbs, and this aspect of their biology is of interest to many researchers—including some at Whitehead Institute. Read the latest story in our Unusual Labmates series to learn more about these fascinating creatures, what Whitehead Institute researchers are hoping to discover by studying them, and why they are worth preserving in the wild.
A strange salamander
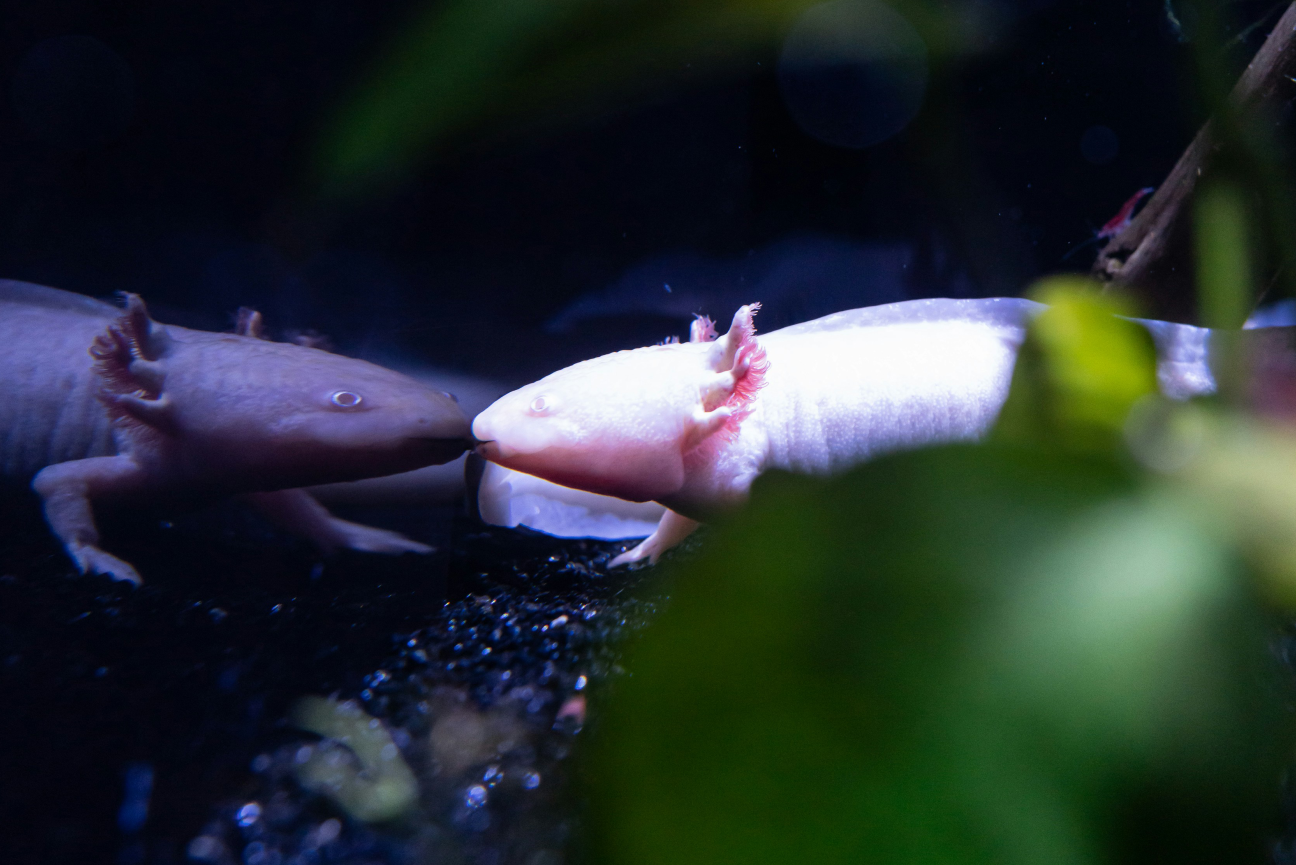
The axolotl's biology makes it a popular pet and a popular model organism for research.
Guillaume de Germain on Unsplash
Axolotls are known for their unusual looks: they appear to be part lizard and part fish, with a long, finned tail good for propelling them through the water, tiny limbs, and a crown of feathery gills like stout ferns wriggling on the sides of their heads. This odd combination of features, plus the wide curved mouth that makes axolotls look like they are always smiling, has charmed people the world over. Axolotls are popular as pets, toys, Halloween costumes, and more.
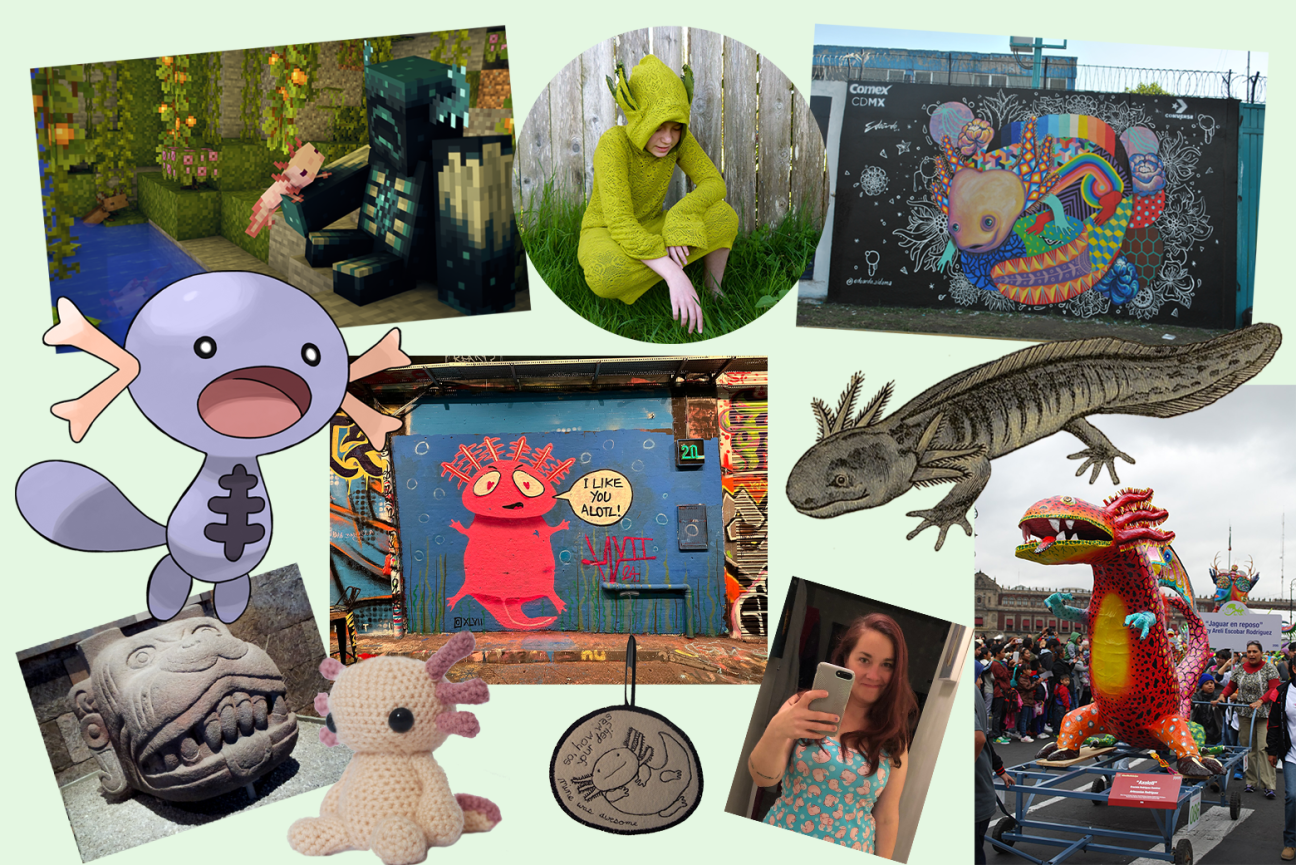
Axolotls appear in art, culture, and merchandise, both in Mexico and around the world.
From left to right. Top row: Axolotl in the game Minecraft (Flowerscow on Deviant Art, CC BY 3.0 DEED); Axolotl dress by Kelly (kellyhogaboom on Flickr, CC BY-SA 2.0 DEED); Mural by Edoardo Aldama (HidroARTE SACMEX, CC BY-SA 4.0). Middle row: Fan art of Wooper, Pokémon inspired by axolotls (TonOfDirt726 on Deviant Art, CC BY 3.0 DEED); I love you Alotl by Matt Brown (londonmatt on Flickr, CC BY 2.0); Axolotl illustration from Appletons' guide to Mexico, by Alfred R. Conkling (public domain). Bottom row: Aztec sculpture of head of the aztec god Xolotl, at Museo Nacional de Antropología de México. (public domain); Crochet axolotl (MaffersToys on Flickr, CC BY-NC-SA 2.0 DEED); axolotl embroidery and axolotl tank top by maggie schreiter (squishythings on Flickr, CC BY-NC 2.0 DEED); “Axolotl” by Graciela Rodriguez Ramirez at the 2015 Monumental Alebrije Parade in Mexico City (Thelmadatter on Wikimedia, CC BY-SA 4.0 DEED).
The axolotls’ unique look is based on some unusual biology. Axolotls are salamanders, a type of amphibian. Amphibians are known for spending part of their lives in the water and part on land, metamorphosing as they grow to switch between traits that favor each ecosystem, like a tadpole turning into a frog. However, unlike most salamanders, axolotls remain in their aquatic juvenile form for their entire lives: permanent tadpoles or nature’s Peter Pans. The axolotl’s closest relative, the tiger salamander, has a more typical life cycle. Tiger salamanders begin their lives in the water, with water-dwelling traits, but they eventually metamorphose into a land-dwelling form. Their adult shape is more conducive to a terrestrial life, with bigger limbs that can support the body on land, a round, finless tail, and bigger lungs to replace their gills.
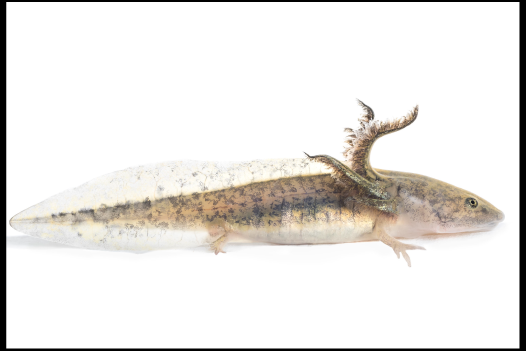
Tiger salamander larvae (left) look similar to axolotls, but they metamorphose into a land-dwelling adult form (right) with different characteristics.
Larval tiger salamander (Ambystoma tigrinum) photographed at Gavins Point National Fish Hatchery by Sam Stukel (USFWS).

"Eastern tiger salamander (Ambystoma tigrinum)" by Peter Paplanus, licensed under CC BY 2.0 DEED
The axolotl’s ancestor shared this metamorphic life cycle, but at some point the species evolved to stay in the water, and so in its juvenile form, for its entire life. The exact causes that drove this evolutionary shift are not known. One contributing factor is that the axolotl’s home waters, a set of lakes in Mexico City, are full of water year-round, whereas many salamanders begin life in vernal pools that dry up seasonally, forcing the salamanders to transition to life on land. It’s also possible that the land around the axolotl ancestors’ lakes was a much worse ecosystem for them than the water, with too many threats or too few resources, making staying in the water a better alternative. Whatever drove the axolotls to make this evolutionary switch, the species now keeps its aquatic lifestyle—and its youthful looks—for life.
The axolotl’s permanent-juvenile form is also highly regenerative. Axolotls can lose and then regrow an entire limb. They can also regenerate parts of major organs including their hearts, livers, eyes, and even brains. These regenerative abilities are what make the axolotl such an interesting subject for researchers such as Whitehead Institute Member Peter Reddien and graduate student in his lab Conor McMann. Reddien studies the biology underlying regeneration across many species, in order to understand its basic principles and mechanisms. Recently, Reddien’s lab has added axolotls to their list of regenerative specimens with a research project led by Conor.
Learning how animals that are able to perform incredible feats of regeneration, such as regrowing entire limbs, are able to do so helps researchers understand the requirements of regeneration. This could inform regenerative medicine strategies to improve human healing or even stimulate regenerative repair.

Graduate student Conor McMann, a member of Peter Reddien's lab, looks at a sample through a microscope.
Madeleine Turner/ Whitehead Institute
Why does an axolotl limb regenerate and a mammal limb doesn’t
Many amphibians have regenerative capabilities, so why have axolotls in particular become the go-to amphibian for regeneration research? Axolotls are easy to breed and maintain in the lab, and easy to collect eggs and embryos from. There are established lines of axolotls with different engineered traits and a stock center, the Ambystoma Genetic Stock Center at the University of Kentucky, that can provide researchers with axolotls that fit their requirements. The axolotl genome is fully-sequenced and lots of tools already exist for experimenting with its gene expression. In large part, the reason for the axolotl’s popularity is that it has become a familiar entity to researchers—a somewhat ironic fate given that at the same time, axolotls have been disappearing from the wild.
Fun fact: axolotls have a huge genome, about ten times larger than the human genome.[2]
Finally, the axolotl’s similarities to humans, rather than its unique features, are another feature that makes it such a good model organism. Other species have greater regenerative abilities; the Reddien lab’s primary model organism, the planarian, for example, is a flatworm that can regrow its entire body from a small remaining piece of the original.
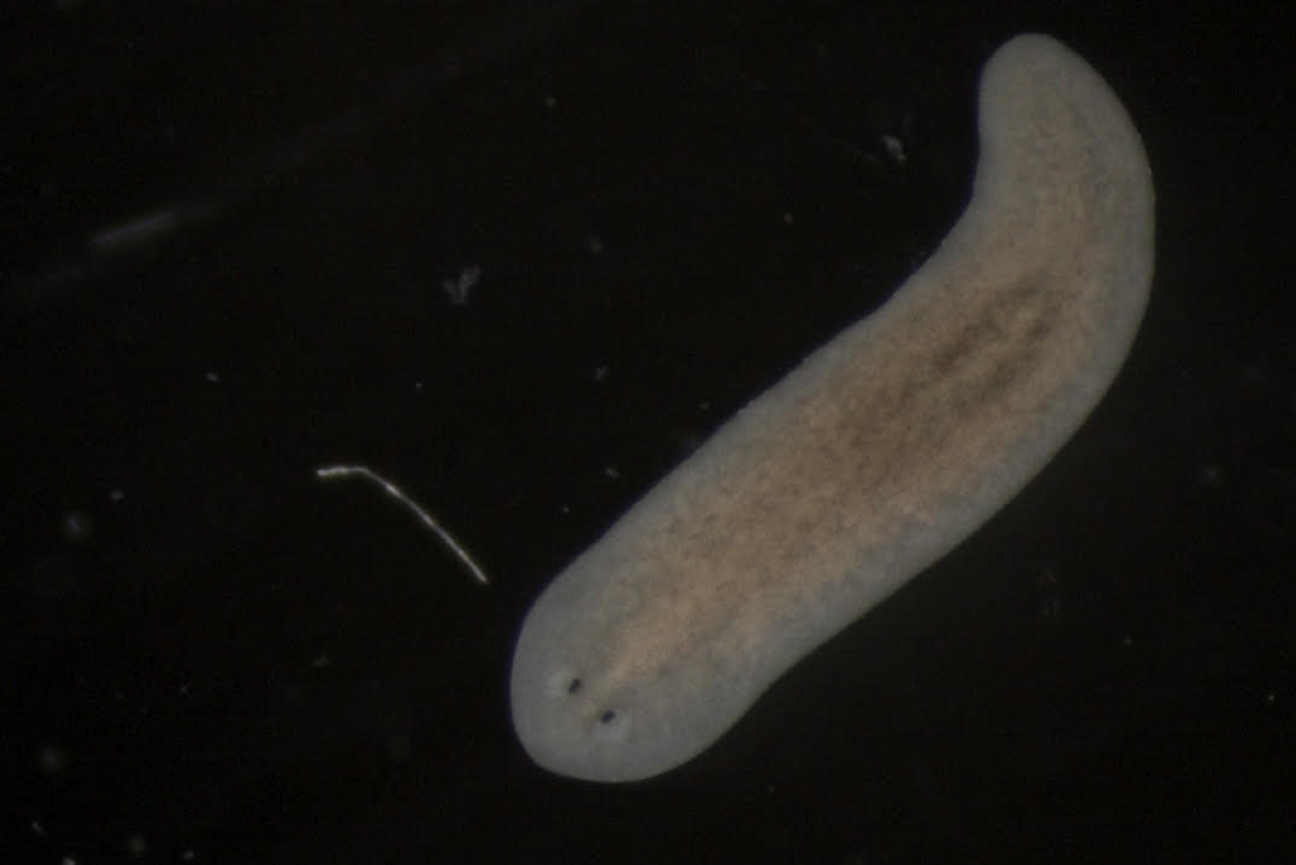
A planarian (Schmidtea mediterranea), the Reddien lab's primary model organism for regeneration research.
Bryanna Isela-Inez Canales and M. Lucila Scimone/ Whitehead Institute
However, most of these species are far removed from humans both on the evolutionary tree and in terms of their body shape. Axolotls are vertebrates (meaning they have a backbone and a skeleton) with four limbs, which makes them good for comparison to humans and mice, the mammal most often used as a model for humans in research. Conor is studying axolotls in comparison to mice: he is looking at the gene expression in the hind limbs of each species after an injury, and searching for certain commonalities. His hope is to understand more about the genes and pathways that axolotls use to regrow a limb, and which of those genes and pathways are still present in mice—which would suggest that they may also be present in humans.
When researchers find that two or more distantly related species rely on the same mechanism to regenerate, this suggests that the mechanism may have been inherited from a shared ancestor; similar, complex traits are more likely to have evolved once in an evolutionary tree rather than in several distinct instances. Therefore, when Conor studies how axolotls regenerate, a large part of what he is looking for is whether they are using genes and biological processes that also exist in some form in mice and humans. The shared mechanisms that Reddien and other researchers have identified across species so far suggest that a distant ancestor of humans—the ancestor that humans share with all bilaterally symmetrical animals—was much more regenerative than we are. If humans retain portions of the pathways that our ancestor used for regeneration, then that may make it more likely that researchers can one day enable some level of regenerative healing in humans, sort of like how it’s easier to recreate a recipe if you have access to most of the original ingredients than if you have to reinvent it with entirely new ingredients.
According to Conor, there are three main ingredients that Reddien lab researchers have observed as needed for regeneration: “You need cells that are capable of rebuilding the missing tissue. You need positional information that is able to direct the proper shape and size of the new tissue as it grows. And you need varying degrees of signaling at the wound site to orchestrate and initiate all these processes.”
The better that researchers can characterize the biology of those three factors in vertebrates like axolotls and mice, the better they will understand what the recipe for vertebrate regeneration is and what ingredients for it are missing in humans. Hopefully, this could one day lead to new strategies for regenerative medicine.
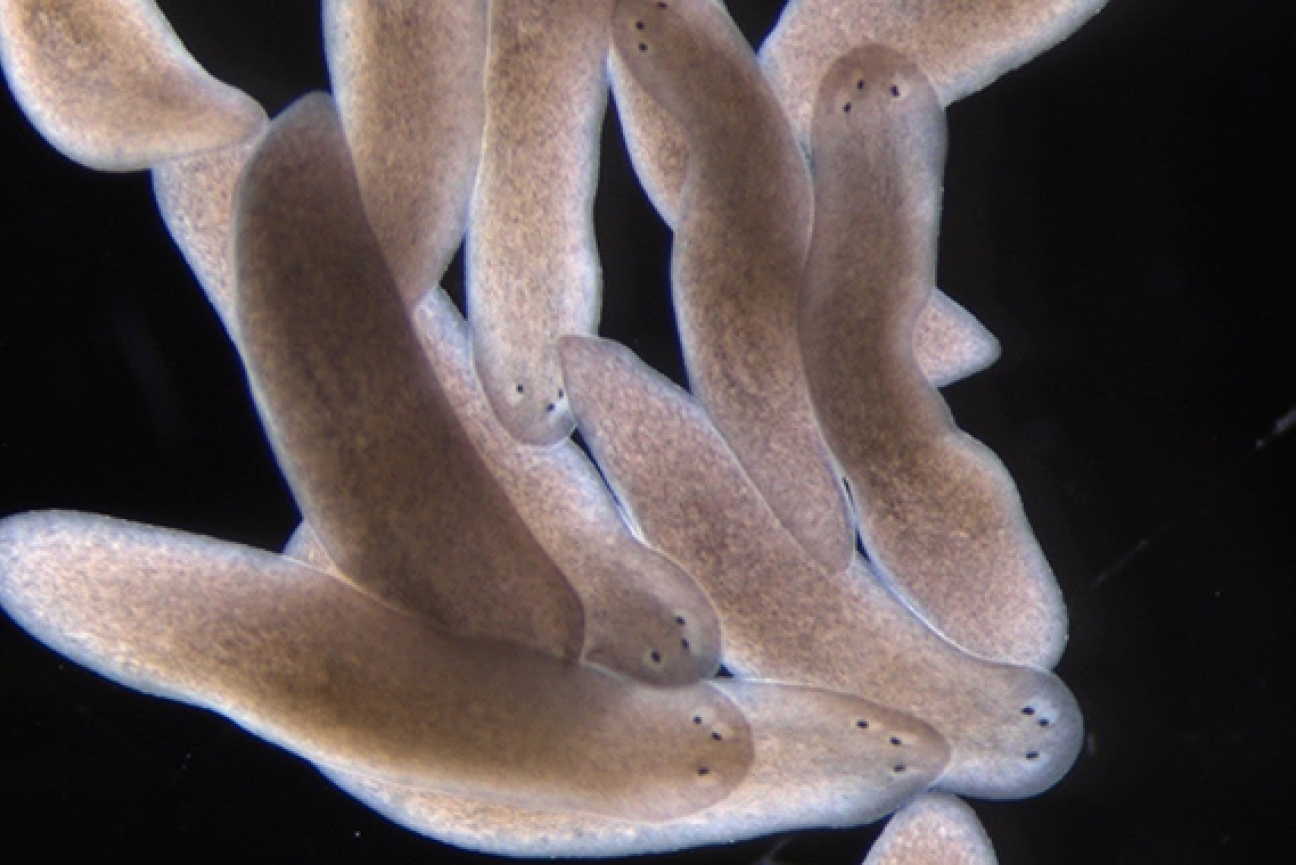
Three-eye planarians generated by a simple surgical trick revealing self-organizing dynamics that occur during regeneration.
Kutay Deniz Atabay/Whitehead Institute
The required ingredient in regeneration that Conor focuses on is positional information, or the information that an organism uses to “remember” the correct blueprint of its body and grow new tissue according to it. All animals use systems of positional information during their development to direct the original formation of their bodies into the correct shape. In planarians, this system stays on for life: Reddien and colleagues have found that certain genes expressed in planarian muscle tissue maintain a GPS system that tells cells where to go and what to become in order to rebuild missing body parts in line with the body’s original shape. This is how, when a planarian loses its tail, the cells repopulating that space know to grow a tail there, rather than a head, as well as what size the tail should be to match the rest of the body. Reddien lab researchers have found that species distantly related to planarians use the same sort of positional information system to guide regeneration, which suggests that such a system was around in the ancestor of most modern animals. There is evidence that vertebrates have retained some aspects of this positional information system.
Conor is now trying to find out how much of the positional information system remains in adult vertebrates. He’s particularly interested in genes that have that pattern in connective tissue cells–connective tissue is the scaffolding, binding, and padding material of the body, like collagen and cartilage–which seem to have taken on muscle’s proposed ancestral GPS role in vertebrates.
To learn more about Conor’s research, watch the video below:
“If there is a robust system of positional information in adult tissues in these organisms, it's not as if regenerative medicine is now easy, but it’s definitely easier,” Conor says. “Adding back into humans a positional coordinate system that can inform the shapes that are required after a wound would be really tricky. Figuring out what we have in terms of a GPS system in our adult tissues won’t immediately lead to regenerative solutions, but I think it will be a prerequisite if limb regeneration is ever going to be possible in humans.”
Rearing axolotls for research
In order to study axolotl regeneration, Whitehead Institute maintains its own colony of axolotls. They live in a connected system of tanks in the aquatic facilities room that are carefully monitored for temperature and water quality. Olivier Paugois manages the aquatic facilities and the care and management of aquatic specimens for the Reddien lab. Most of his charges are fish, but the axolotls have been a welcome addition.
“The fun of working in aquatics is that there is such a diversity in the species, so you are always learning something new. Establishing this axolotl colony has been an interesting new challenge,” Olivier says.
Olivier’s first challenge with the axolotls was to retrofit a fish system for them to live in. He did this by replacing the smaller fish tanks with larger tanks—axolotls can grow up to a foot long[3]—and adding a chiller to the existing filtration systems to keep the water at a cool 16 degrees Celsius (60.8 Fahrenheit). Axolotls are poikilotherm, meaning they cannot regulate their own temperature, and they can get stressed or sick at higher temperatures.
Each axolotl must be kept in its own tank, because they are territorial and will attack each other if kept in the same small space. However, the water in each tank is part of the same circulating system, which goes through four types of filtration to remove waste and keep the water clean. Olivier monitors the axolotls’ behavior to make sure they are healthy. The main things he looks for are whether they are interested in their food during feeding time and whether they have their gills up and active. Axolotls use their gills to breathe by moving them around to make water pass through them and pulling oxygen from the water. (Axolotls can also breathe in other ways, including through their skin or using their lungs to breathe air – but they have small, underdeveloped lungs that are not as useful as the lungs in salamanders that metamorphosize into land dwellers.)
“I like when they look happy. If that’s not important to you, then aquatics is the wrong career,” Olivier says.
Axolotls in the wild
Axolotls were originally found in a series of lakes in Mexico City, Mexico. The axolotl has an important cultural history in Mexico. Its name comes from the god Xolotl, who according to Aztec mythology hid himself in salamander form to avoid being sacrificed.[4] Axoltols have continued to appear in Mexican art and culture ever since. Today they are even represented in Mexico’s currency, on the back of the 50 peso bill.[5]
If the species is so beloved, and so prevalent in research labs and pet owners’ homes, why is it critically endangered in the wild? The problem is not with the animal: axolotls are very good at reproducing, and when kept in captivity will quickly increase their numbers. The problem is that the axolotl’s natural habitat is disappearing and becoming less hospitable. Most of Mexico City’s historic lakes are long gone due to human activity and environmental degradation. The lakes have been drained and diverted over the course of centuries to prevent flooding of human infrastructure in the low-lying valley city, as well as for irrigation. The axolotls’ habitat has dwindled to a series of canals that are what remains of one of the lakes, Lake Xochimilco.

One of the Xochimilco canals, the last remnants of the axolotls' natural habitat.
"Un canal. Xochimilco (Ciudad de México, México)" by LBM1948 is licensed under CC BY-SA 4.0.
The wild axolotl population has experienced a swift decrease in recent decades. A survey of the Xochimilco canals done in the 1990s found thousands of axolotls per square kilometer, while a 2014 follow up found only 35 per square kilometer.[6] Threats to their existence include pollution and invasive predators in the canals.[7] However, many people are working to rebuild the axolotl population. A large restoration effort led by researchers at the National Autonomous University of Mexico has attracted global attention and support.[8,9]
Although the axolotl is growing in popularity around the world, the heart of the effort to save them remains—and must remain—in Mexico City. The lakes there are the axolotls’ only natural habitat, and the individual axolotls found there are “wild type,” meaning they retain the genetics of the original wild population. The majority of axolotls found as pets or in research labs are actually hybrids, which have been interbred with related salamander species such as the tiger salamander.[10,11] This interbreeding helped to increase genetic diversity in the captive axolotl population. However, the hybridization also means that if people want to preserve the wild axolotl, they can only work with axolotls from the Xochimilco canals and cannot reintroduce research or domestic specimens into the wild.
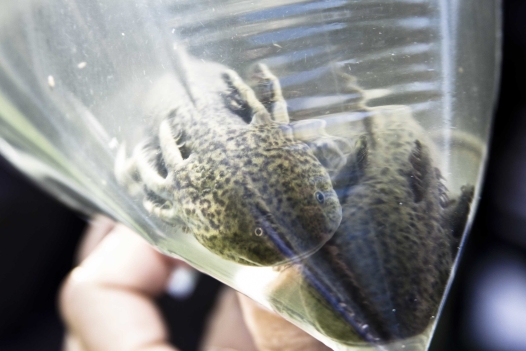
The Secretariat of Environment and Natural Resources of Mexico (Semarnat) prepares to release axolotls into the waters of Xochimilco.
Semarnat

Semarnat
Conservation of wild axolotls is not immediately relevant to Conor and Peter’s research. Whitehead Institute has a thriving colony of axolotls, and their tiger salamander genes do not prevent them from being good models for regeneration research. Nonetheless, preserving biodiversity is an important cause to many biology researchers, both for the sake of the species and the ecosystems at stake and for the sake of future research.
“The diversity of biology that exists in the animal kingdom is so vast, and when that diversity is put at risk and we lose access to studying it, that can really limit what we are able to understand about the full spectrum of biological phenomena,” Conor says. “There are a handful of model organisms that have been really carefully characterized and are commonly used, like mice and fruit flies, but as soon as you start getting outside of the traditional model organisms, you can quickly see that biology is capable of a lot more than can be characterized in those few. When you come across an organism like the axolotl that is so strange and so uniquely capable of a lot of regenerative feats, it makes you wonder what other kinds of biological phenomenon you're losing insight into when species go extinct.”
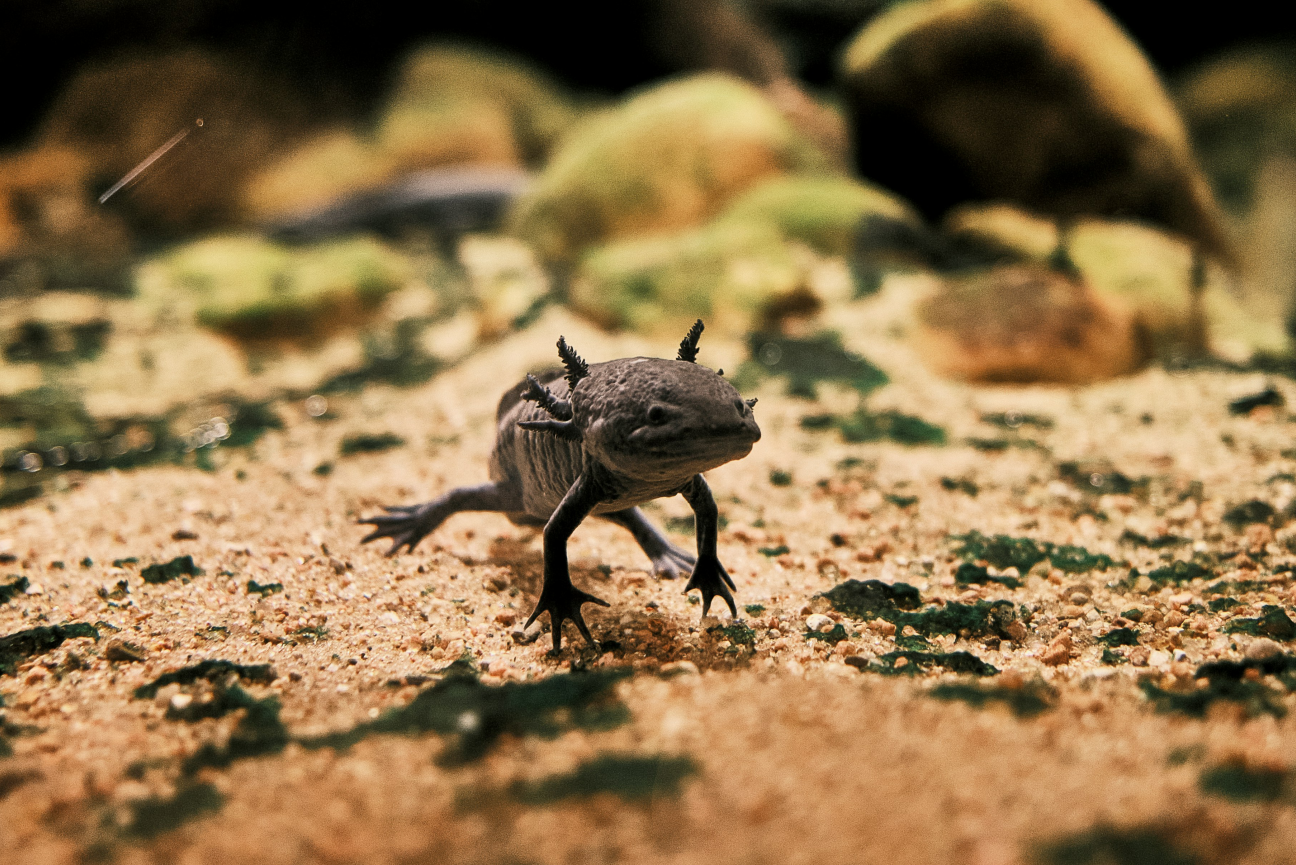
"When you come across an organism like the axolotl that is so strange and so uniquely capable of a lot of regenerative feats, it makes you wonder what other kinds of biological phenomenon you're losing insight into when species go extinct.” - Conor McMann
Nathan Guzman/ Unsplash
Citations:
1. IUCN SSC Amphibian Specialist Group (IUCN SSC). (2019, October 23). The IUCN Red List of Threatened Species. IUCN Red List of Threatened Species. https://www.iucnredlist.org/species/1095/53947343
2. Nowoshilow, S., Schloissnig, S., Fei, J.-F., Dahl, A., Pang, A. W., Pippel, M., Winkler, S., Hastie, A. R., Young, G., Roscito, J. G., Falcon, F., Knapp, D., Powell, S., Cruz, A., Cao, H., Habermann, B., Hiller, M., Tanaka, E. M., & Myers, E. W. (2018). The axolotl genome and the evolution of key tissue formation regulators. Nature, 554(7690), 50–55. https://doi.org/10.1038/nature25458
3. National Geographic. (n.d.). Axolotl. National Geographic. https://www.nationalgeographic.com/animals/amphibians/facts/axolotl
4. Ibid
5. Mexico, B. of. (n.d.). 50-peso banknote (G-type). Banco de Mexico. https://www.banxico.org.mx/banknotes-and-coins/50-peso-banknote-g-circu…
6. Smith, J. E., & Rojas, L. A. (2023, December 5). What it takes to save the axolotl. The New York Times. Retrieved March 18, 2024, from https://www.nytimes.com/2023/12/05/science/mexico-axolotl-biology.html
7. Vance, E., & Nature Magazine (2017, November 20). Biology's Beloved Amphibian--the Axolotl--Is Racing toward Extinction. Scientific American. Retrieved March 18, 2024, from https://www.scientificamerican.com/article/biologys-beloved-amphibian-t…
8. Smith, J. E., & Rojas, L. A. (2023, December 5).
9. Laboratorio de Restauración Ecológica UNAM. LRE UNAM. (n.d.). https://www.restauracionecologica.org/
10. Motivans, E. (2023, June 15). The axolotl: the salamander that defies science. ZME Science. https://www.zmescience.com/feature-post/natural-sciences/animals/amphib…
11. Woodcock, M. R., Vaughn-Wolfe, J., Elias, A., Kump, D. K., Kendall, K. D., Timoshevskaya, N., Timoshevskiy, V., Perry, D. W., Smith, J. J., Spiewak, J. E., Parichy, D. M., & Voss, S. R. (2017). Identification of mutant genes and introgressed tiger salamander DNA in the laboratory axolotl, Ambystoma Mexicanum. Scientific Reports, 7(1). https://doi.org/10.1038/s41598-017-00059-1
Video producer: Madeline Turner
Contact
Communications and Public Affairs
Phone: 617-452-4630
Email: newsroom@wi.mit.edu