Unusual Labmates: Fruit flies
All the buzz in the lab
On a sunny summer morning in Cambridge, Massachusetts, Mariyah Saiduddin walked into a room and was met by the sight of thousands of fruit flies. For most people, this would be an emergency: time to call an exterminator, take out the trash, and scrub the room from top to bottom. However, this room full of flies is part of Whitehead Institute Director Ruth Lehmann’s lab, where fruit flies are seen not as pests but as valuable research tools—and are safely contained in vials. Saiduddin is a graduate student researcher in Lehmann’s lab who uses a fraction of the flies in the room in her research.
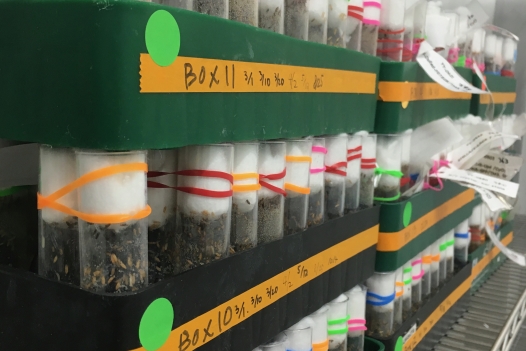
Vials of flies.
Jaclyn Fingerhut/ Whitehead Institute
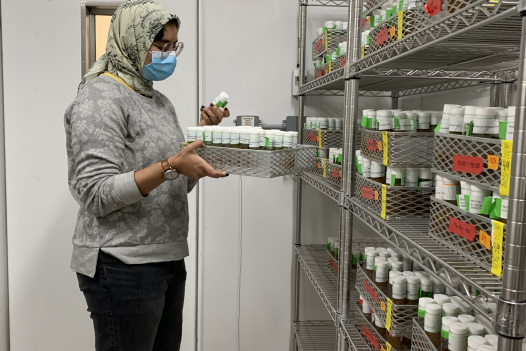
Mariyah Saiduddin in the stock room, where the lab’s common fly stocks are stored.
Courtesy of Mariyah Saiduddin/ Whitehead Institute
The flies found in Lehmann’s lab, and in the adjacent lab run by Whitehead Institute Member Yukiko Yamashita, are not exactly like their less-beloved wild counterparts. Fruit flies have been used in research for more than a century, and in that time, they have been engineered to become powerful, malleable models capable of answering questions in many areas of research. The most common species used in research is Drosophila melanogaster, often referred to simply as “Drosophila.” The researchers who use flies call themselves Drosophilists, and their community around the world works together to maintain a rich variety of flies and create new tools with which to manipulate those flies. In the past century, work in fruit flies has led to six Nobel Prizes in Physiology or Medicine, and has shed light on topics from the basics of genetics, to the principles of embryonic development, to circadian rhythms, to the immune system, to a plethora of diseases.

“Drosophila melanogaster - side (aka)” by André Karwath is licensed under CC BY-SA 2.5.
A very fly model organism
Fruit flies became a go-to research tool during the explosion of genetics research around the turn of the 20th century. What makes them such a good model organism? First of all, they are easy and relatively cheap to raise in large numbers. They have short lifespans and quick reproduction times, so researchers can rapidly breed and study multiple generations. Fruit flies are ready to reproduce—growing from embryo to larva to adult—in under two weeks and then can lay hundreds of eggs in a matter of days.

An agar plate full of fly eggs. Flies are kept overnight in a collection cage with an agar plate at the bottom. By the next day, they will have laid hundreds of eggs in the agar.
Ruoyu Chen/ Whitehead Institute
Fruit flies and humans have enough similarities in their genetics and development that research in fruit flies often reflects human biology. In particular, when it comes to genetics, fruit flies have more commonalities with humans than they have differences. Nearly three-quarters of the genes that cause diseases in humans have an equivalent gene—one derived from the same ancestral gene—in fruit flies.[1]
Fun fact: Researchers who work with fruit flies often get to name the genes whose function they discover, and they like to get creative. Lehmann named a gene oskar after the main character in the novel The Tin Drum. A mutation to the gene prevents the embryo from segmenting properly and so makes embryos short and unable to mature, like the character in the book, who refuses to become an adult. Researchers in the Lehmann and Yamashita labs say that some of their favorite fly gene names include fuzzy onions, string of pearls, hiphop, and bag of marbles.
Not only are fruit flies naturally suited for research, but over the years they have been engineered to become even better research subjects. One of the earliest improvements to fruit flies as a research model came from researchers discovering that they could create flies with genetic mutations that change things like a fly’s eye color, wing shape, body shape, or the bristles on its thorax. Researchers began selectively breeding different lines of fruit flies to have these distinctive physical traits or “markers,” which make them easy to tell apart.
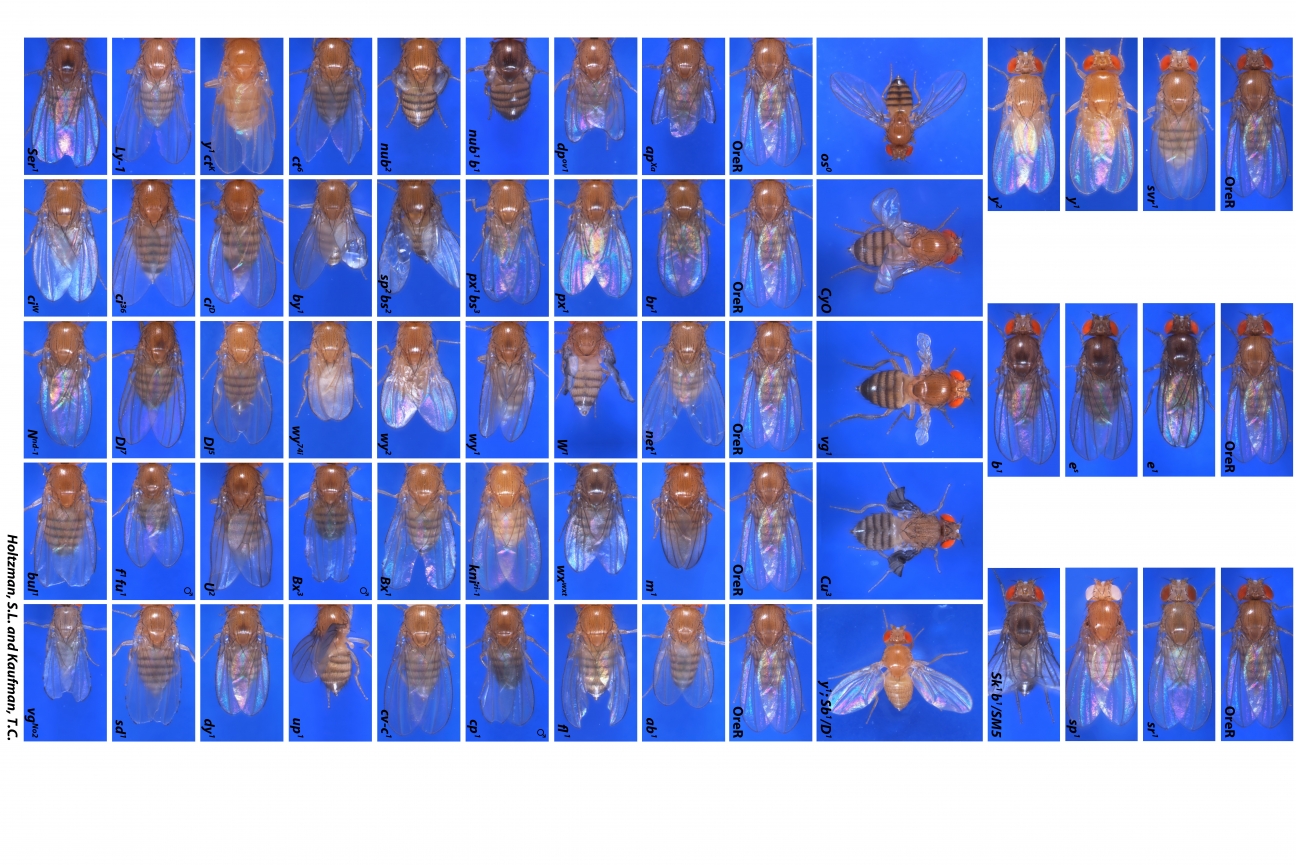
Drosophila body color and wing mutants
Courtesy of Thomas Kaufman and FlyBase.org.

Drosophila eye color mutants. Fly researchers can order fruit flies with any of these common genetic markers (and many more) from stock centers like the Bloomington Drosophila Stock Center in Indiana. The flies get sent in vials by regular mail.
Courtesy of Thomas Kaufman and FlyBase.org.
Researchers can tie a visible genetic marker, such as curly wings, to a genetic mutation that they are studying that may not be visible, so that they can easily sort their flies. For example, Whitehead Institute researchers using flies to study mutations that affect the germ cells, the set of cells that make or become eggs and sperm, cannot tell just by looking at a fly whether it has a mutation that affects germ cells, but if they tie inheritance of the mutation to the curly wing marker, then the flies with the desired mutation become easy to identify.
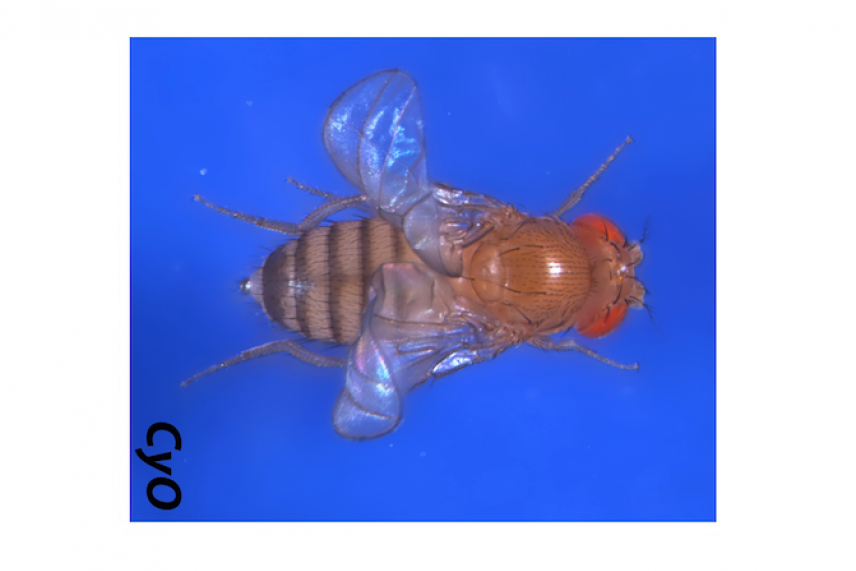
Courtesy of Thomas Kaufman and FlyBase.org.
The photo above shows the curly wing mutation. In the video below, a fly with the mutation grooms itself in Yamashita’s lab.
Jaclyn Fingerhut/ Whitehead Institute
Drosophila biologists have adapted tools from other model organisms to control and study essentially any gene in flies. The Gal4-UAS system, which was developed based on a gene and gene regulator found in yeast, is now commonly used in flies. The system lets researchers activate a gene only in certain tissues or sets of cells. For example, researchers may want to know what part of the brain a certain gene is active in, so they will use the Gal4-UAS system to express green fluorescent protein (GFP) only in cells that would also normally express the gene of interest, allowing researchers to map gene activity based on fluorescence. Or, Gal4-UAS can be used to turn off a gene involved in embryonic development, to see what changes when that gene is not active. This is a common approach that researchers use to figure out a gene’s function; it works in the same way that one could deduce the purpose of brakes on a car by taking the brakes off and observing the car being unable to stop when its driver steps on the brake pedal. Gal4 lines can be developed and tested quickly, in a couple of months, and because the Drosophilist community tends to share their resources, as soon as one lab has developed a Gal4 line for a gene, any fruit fly lab can use that tool to ask their own research questions. Thousands of Gal4 lines are maintained in centralized collections, making them very easy to access.

An example of Gal4 at work. Nuclei are in blue, and GFP is in green. Mariyah Saiduddin is studying what happens in early embryos when a certain gene is over-expressed. She used GAL4 to activate expression of that gene during development of the egg so that its protein product would be present in the early embryo, and tagged the gene's DNA sequence with GFP so that the protein it produces would be easy to see under the microscope. In this image of a 3-hour old embryo, in which the primordial germ cells have already formed (on the right), the protein (in green) is being localized to the membrane. Saiduddin says that this research will help her understand how primordial germ cells form in the early embryo.
Mariyah Saiduddin/ Whitehead Institute
The embryonic days of fly research
The transformation of fruit flies from wild pests into top notch research tools began in the early 1900s. Charles W. Woodworth, at Harvard University at the turn of the 20th century, is credited with being the first researcher to breed Drosophila in large numbers and with suggesting that the species could be used to study genetics, then a new field of research.[2] Thomas Hunt Morgan, at Columbia University, was one of several researchers to follow Woodworth in using fruit flies for his research, and it was Morgan who really established fruit flies as a model organism, through both his own success and that of the students who came out of his lab and the soon-famous Columbia fly room.

Thomas Hunt Morgan in the Columbia fly room.
Courtesy of the American Philosophical Society
For his research, Morgan bred fruit flies until one developed a mutation, white eyes (most fruit flies’ eyes are red), and then continued breeding the mutant and its descendants to track patterns in inheritance of the white-eyes trait. With these experiments, Morgan showed that genes, which had recently been established as the smallest units of inheritance, are organized on chromosomes, cellular structures each one of which contains a certain, consistent set of genes. One of Morgan’s students, Alfred Sturtevant, expanded on this work, showing that the genes on each chromosome can be mapped in a specific linear order. The proof of the chromosome theory of inheritance won Morgan the 1933 Nobel Prize in Physiology or Medicine and the mutation that Morgan identified, white, is still used as a marker in fruit flies today. Morgan and the scientists who came through his lab continued to do groundbreaking research, demonstrating the potency of fruit flies as a model, and soon flies became a popular research tool.
Research in fruit flies has led to five further Nobel Prizes since Morgan’s, including the 1995 prize awarded to Edward B. Lewis, Christiane Nüsslein-Volhard and Eric Wieschaus for their discoveries regarding “the genetic control of early embryonic development.” These three researchers identified and discovered the function of key genes involved in determining and carrying out the blueprint for a fly’s body during development. Nüsslein-Volhard and Wieschaus systematically mutated many flies in order to discover the genes involved in body patterning. After they introduced lots of mutations, they observed what happened to the flies, and then they determined which genes had been mutated to cause the effects to body patterning that they observed. Using this strategy, they identified and characterized many key genes involved in guiding the development of an embryo into a segmented body.

Larvae with mutations that affect their segmentation, from Christiane Nüsslein-Volhard's Nobel Lecture. The middle larva is normal. The top larva has a mutant paired gene and so is missing every other segment, leaving it with around half the normal number of segments. The bottom larva is a knirps mutant, and so is missing most of its abdominal segments.
Courtesy of © The Nobel Foundation, https://www.nobelprize.org, from Christiane Nüsslein-Volhard's Nobel Lecture.
Lewis, meanwhile, identified and determined the function of what would come to be known as homeotic genes, the genes that determine which specific body parts grow in each body segment: these genes essentially determine the blueprint for the fly’s body, and—as Lewis showed—when mutated lead to some very unusual body plans. Collectively, these researchers’ discoveries illuminated both the genetics and the evolution of the body plan in flies—work that was quickly extrapolated to other species, including humans, whose development occurs in a similar fashion.

Lewis’ experiments with the bithorax complex, one of the two main sets of homeotic (body patterning) genes in Drosophila, led to a mutant fly with two thoracic segments and therefore four wings.
Courtesy of the Caltech archives.
Lewis, Nüsslein-Volhard and Wieschaus’ work set the stage for future researchers such as Lehmann and Yamashita who study development in flies. In fact, Nüsslein-Volhard had a direct influence on Lehmann: Lehmann trained with her as a graduate student. Nüsslein-Volhard’s later work provided important insights into the morphogen gradients that help guide the developing embryo in assembling itself correctly, and included an in-depth gene screen of zebrafish using the same extensive process of mutation and observation that she and Wieschaus had used in Drosophila.
Fly research spreads its wings at Whitehead Institute
Researchers at Whitehead Institute are using fruit flies to answer a wide variety of questions. In previous years, former Whitehead Institute Member Terry Orr-Weaver used Drosophila to study the process of cell division during development. She looked at questions such as what determines cell size, what regulates the transition from egg to embryo, and how DNA is accurately replicated and sorted into dividing cells. Whitehead Institute Member David Bartel, also a professor of biology at the Massachusetts Institute of Technology (MIT) and an HHMI investigator, studies RNA and has done some of that research in flies. His work has improved our understanding of how tiny regulatory RNAs called microRNAs target and initiate the destruction of the RNAs that code for proteins in many species, including flies. He used these insights to create TargetScanFly, a database that provides researchers around the world with fly microRNAs’ predicted targets. Bartel’s lab also recently discovered how some microRNAs are rapidly degraded in Drosophila cells and how other types of small regulatory RNAs are protected from this degradation. In other studies of gene regulation in flies, performed in collaboration with Orr-Weaver, the Bartel lab identified the RNAs that are first produced by the developing embryo and determined why RNAs of some genes are much better than those of others at producing proteins in fly oocytes and early embryos.
Lehmann and Yamashita use fruit flies to study germ cells, the cells set aside to make or become eggs and sperm—as did Orr-Weaver. The germ line is set aside from the rest of the body’s cells early on, and has the rare property of being, essentially, immortal: all of the other cell lines in the body will eventually die with it, but germ cells survive to become offspring, which contain new germ cells, and so on through the generations.
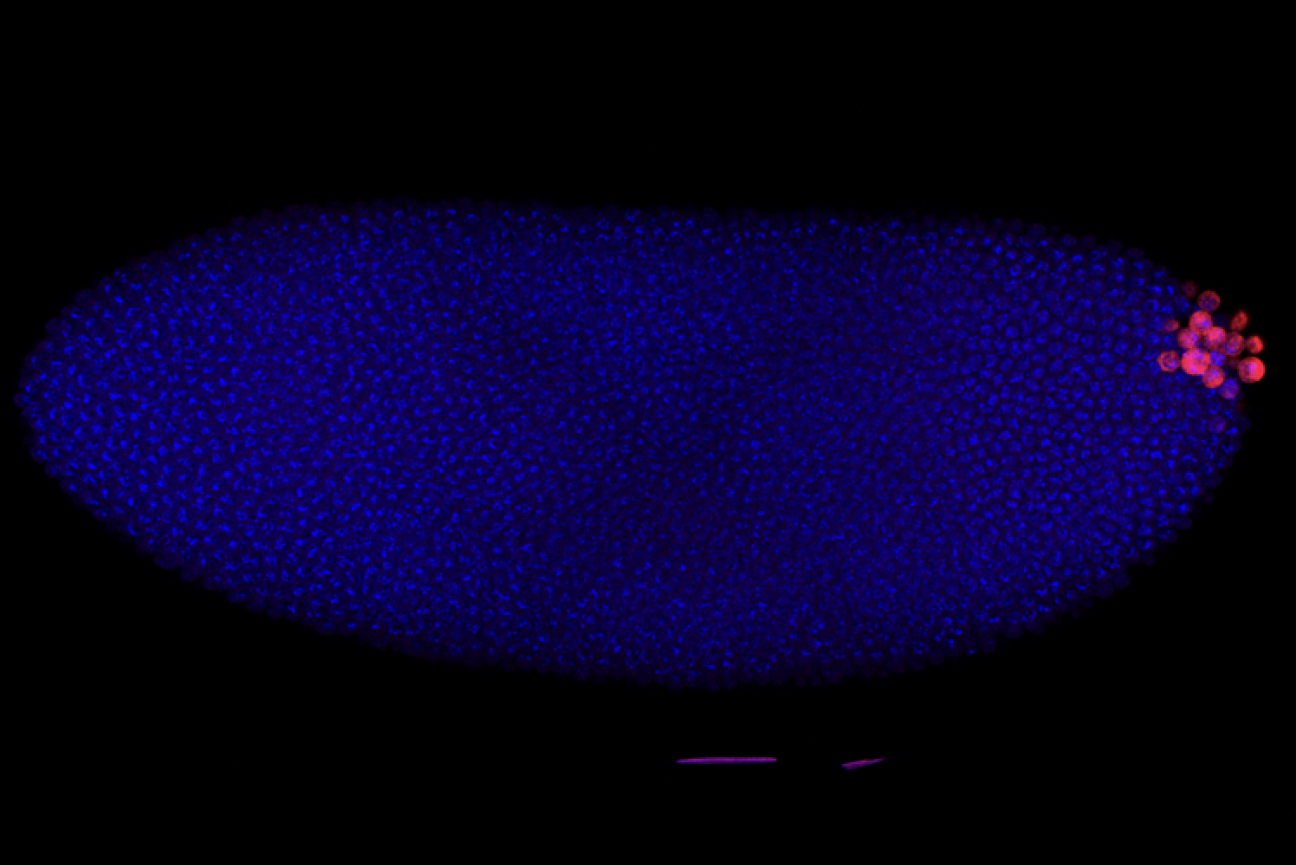
A Drosophila embryo with DNA stained in blue and germ cells stained in red. The germ cells are the first cells to form in a new embryo.
Ruoyu Chen/ Whitehead Institute
Lehmann, who is also a professor of biology at MIT, studies how germ cells are set aside, how they migrate during development, and how the germ line is maintained into adulthood. During development of the gonads, cells must work in perfect sync. They follow cues, many of which are still unknown, to ensure that every cell—including germ cells—ends up in the right place at the right time to form functioning, fertile ovaries or testes. Lehmann’s research has shed light on how these processes occur. Lehmann’s lab also studies how RNA is regulated and organized within germline-specific granules inside of the cells. Another interest of the lab is the inheritance of mitochondria, structures inside of cells that provide energy, which are passed down through the generations exclusively through the female germline. Mitochondria carry their own genomic DNA, and this could accumulate deleterious mutations over time; Lehmann’s lab has helped to determine how the germline manages to selectively inherit mitochondria that are mutation-free or healthy.

A Drosophila embryo with germ cells in magenta, nuclei in blue, and cell membrane in white. Some nuclei are not encased in a cell membrane yet. Only the germ cells have formed at this stage.
Mariyah Saiduddin/ Whitehead Institute
Yamashita, who is also a professor of biology at MIT and an investigator with the Howard Hughes Medical Institute, studies many aspects of germ cell biology in the context of the adult Drosophila testis. Germline stem cells divide asymmetrically, so one dividing stem cell produces one differentiated cell that will go on to become sperm and one new stem cell that will replenish the germline. Yamashita’s lab has studied what distinguishes the cellular components that are inherited by the new stem cell versus the differentiating cell, as well as how the stem cell is able to identify and retain those components. If this asymmetrical division goes awry, then the germline could be lost. Yamashita’s lab also studies a type of repetitive DNA, which contains many repeats of the same sequences of nucleotides (DNA building blocks) that do not code for any genes. This genetic material was once considered “junk DNA.” They found that this “junk” actually helps to ensure that each germ cell contains the proper number of chromosomes, which is necessary in order to produce viable offspring. The lab continues to look at how repetitive DNAs are maintained and at their roles in germ cell development.
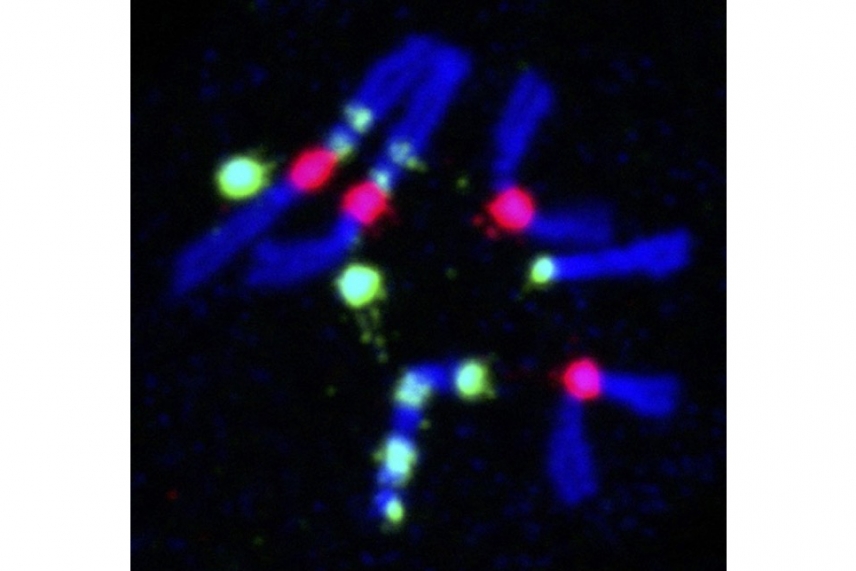
Repetitive DNA in Drosophila chromosomes. DNA is stained in blue, and red and green show two different repeats.
Courtesy of Yukiko Yamshita/ Whitehead Institute
Fortunately for researchers in Lehmann and Yamshita’s labs, the fly research world is one that promotes resource sharing. As they seek to better understand development and related topics, Whitehead Institute researchers have many rich resources to draw from. FlyBase is an online database of Drosophila genetic and molecular data, which contains the complete annotated Drosophila melanogaster genome. The site also has educational resources, community networking links, images and videos, and more. Fly researchers can order the flies they need from stock centers that maintain thousands of lines with different genetic variations, suitable for different research questions. Two of the main stock centers are in Bloomington, Indiana and Kyoto, Japan.
“The fly community, from the very beginning on, has been an example of sharing before publication and exchanging tools and ideas, which is how the best science happens,” Lehmann says.
Fly researchers regularly gather at meetings such as the Annual Drosophila Research Conference, regional Drosophila meetings, and the biennial Crete meeting for principal investigators—which has been held every other year for more than four decades—to exchange ideas and to foster trust and collaboration in the community.
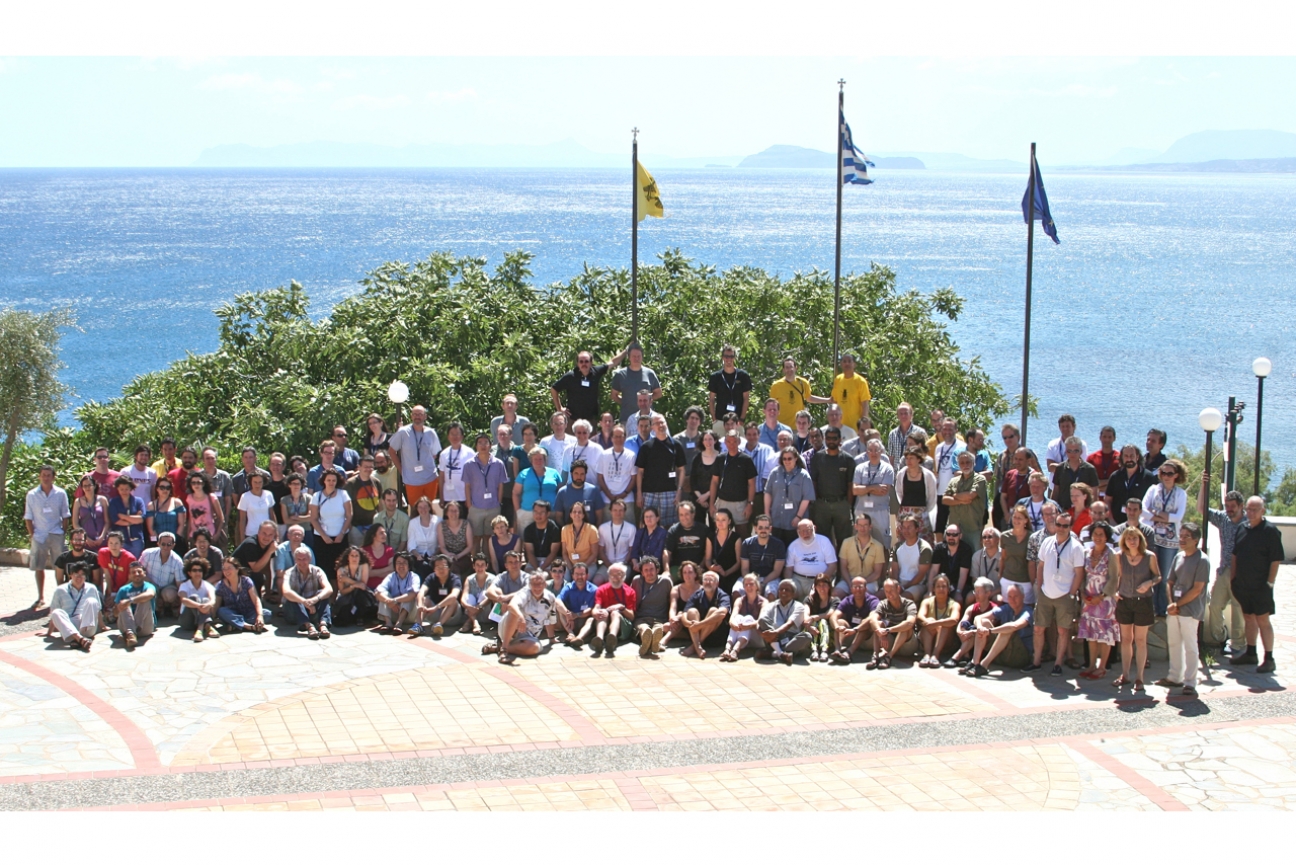
Attendees at the Crete meeting
Courtesy of Ruth Lehmann
In the typical communal spirit of Drosophilists, Lehmann and Yamashita have physically joined their labs and share a fly room—a room full of microscopes and tools for examining the fruit flies. In order to pursue their inquiries, researchers in both labs allocate a portion of their time to an important activity: keeping their flies alive and breeding.
Drosophilist: A day in the life
Each researcher in the lab keeps their own stock of flies. The flies live in vials that are partially filled with wet, packed down food—typically a mixture of yeast, cornmeal, agar and a few other ingredients. The tops of the vials are sealed with cotton swabs.
Jacklyn Fingerhut/ Whitehead Institute
Adult flies rest on the sides of the vial, and lay their eggs in the food. Larva hatch and live in the food mixture as they grow through several molts. Eventually, they crawl out of the food and form pupae on the sides of the vials. In a few days, adult flies emerge from the pupal casings and soon begin to mate, continuing the cycle.
A regular part of work in a fly lab is “flipping flies,” or transferring flies into a new vial with fresh food. This has to be done regularly to keep the vials from overcrowding as the fly population expands. Researchers in fly labs soon become adept at flipping vials.
Flipping flies into new vials
Jaclyn Fingerhut/ Whitehead Institute
Researchers also flip flies or clear vials of adults in preparation for breeding specific crosses. If a researcher wants to make sure that a specific line of females flies mates with a specific line of males, then they need to use virgin females. That’s because female flies can store sperm and use it to fertilize their eggs later, so the offspring of a non-virgin female could be from the male she just mated with or from a male she mated with as long as two weeks ago.
Fun fact: although fruit flies are tiny, their sperm are some of the largest in the animal kingdom. One species of Drosophila, Drosophila bifurca, has the longest known sperm of any animal: its sperm can reach roughly 6 centimeters in length when uncurled, which is 20 times longer than the fly itself.[4]
The most common way for researchers to ensure that the females they use for breeding are virgins is to clear a vial of all of its adult flies, and then wait for new adults to hatch out of their pupal casings. Adult flies cannot mate for the first few hours of their lives, and if kept in chilly conditions—18 degrees Celsius—then they cannot mate for 18 hours. Therefore, a researcher can clear a vial of adult flies, leave it for up to 18 hours, and then collect all of the adult females from the vial, confident that they are virgins.

Jaclyn Fingerhut, a postdoc in Yamashita’s lab, uses Drosophila melanogaster to study how the sperm tail is assembled, which allows sperm to travel to an egg to fertilize it. This image shows a group of immature sperm that will develop synchronously, with spermatid nuclei (in white) clustering on the left. The sperm tails will grow to the right. Two different mRNAS important for the assembly of the sperm tail are shown in red and cyan.
Jaclyn Fingerhut/ Whitehead Institute
Taking a closer look
When the researchers want to get a close-up view of their specimens, they take them out of the vials and examine them under the microscope. In order to do this, they dose the flies with carbon dioxide (CO2), which keeps them asleep on a pad under the microscope.

The CO2 flowing through this pad causes fruit flies to become unconscious, so they can be examined under the microscope.
Jaclyn Fingerhut/ Whitehead Institute
Researchers use gentle tools to maneuver the unconscious flies. The Lehmann lab uses one type of common fly mover: paintbrushes. The Yamashita lab uses another: feathers.

Flies being sorted on a CO2 pad with a feather, which is taped inside a pipette for better control
Jaclyn Fingerhut/ Whitehead Institute
Each lab member has their own brush or feather that feels best in their hands—the Yamashita lab also selects their feathers based on color.
“You learn everyone’s favorite color quickly,” Fingerhut says—hers is purple. Most of the feathers are of the craft store variety, but Yamashita uses real bird feathers (sanitized for lab use).
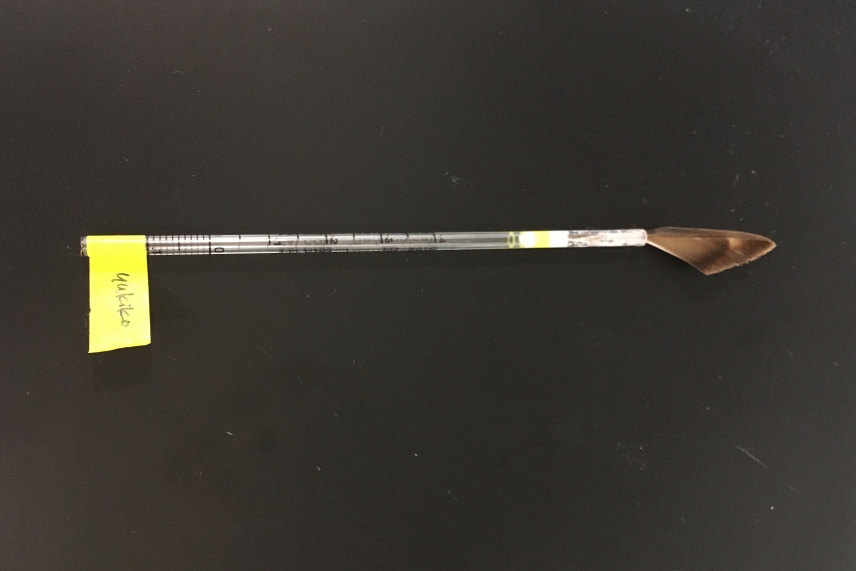
Yukiko Yamashita’s feather
Jaclyn Fingerhut/ Whitehead Institute
Under the microscope, it’s very easy to tell the flies apart by their genetic markers. It’s also possible to view the stages of fruit fly development from start to finish.
“In the stages that we're studying, the embryo will undergo massive morphological changes that you can watch happen over a few hours using video imaging. There's just so much of what’s going on during those few hours that we don't know anything about, but you can see it with your own eyes easily with the microscope,” Saiduddin says.
In the following video, Saiduddin captured germ cell precursors forming in a Drosophila embryo. They appear in the posterior pole, shown on the right. Images were captured every 30 seconds.
Credit: Mariyah Saiduddin/ Whitehead Institute
“When you look at the testis or the ovary, you can see single cell resolution, so you can see what's happening in all the different cell types, kind of all at once, and you can get a picture of what genes are important at what stage of germ cell development,” Fingerhut says. “You can see the whole process going on in one snapshot when you look at a single tissue, like you can see the stem cells, and their niche, and then you can see every stage up until a mature sperm that's ready to go on to fertilization.”
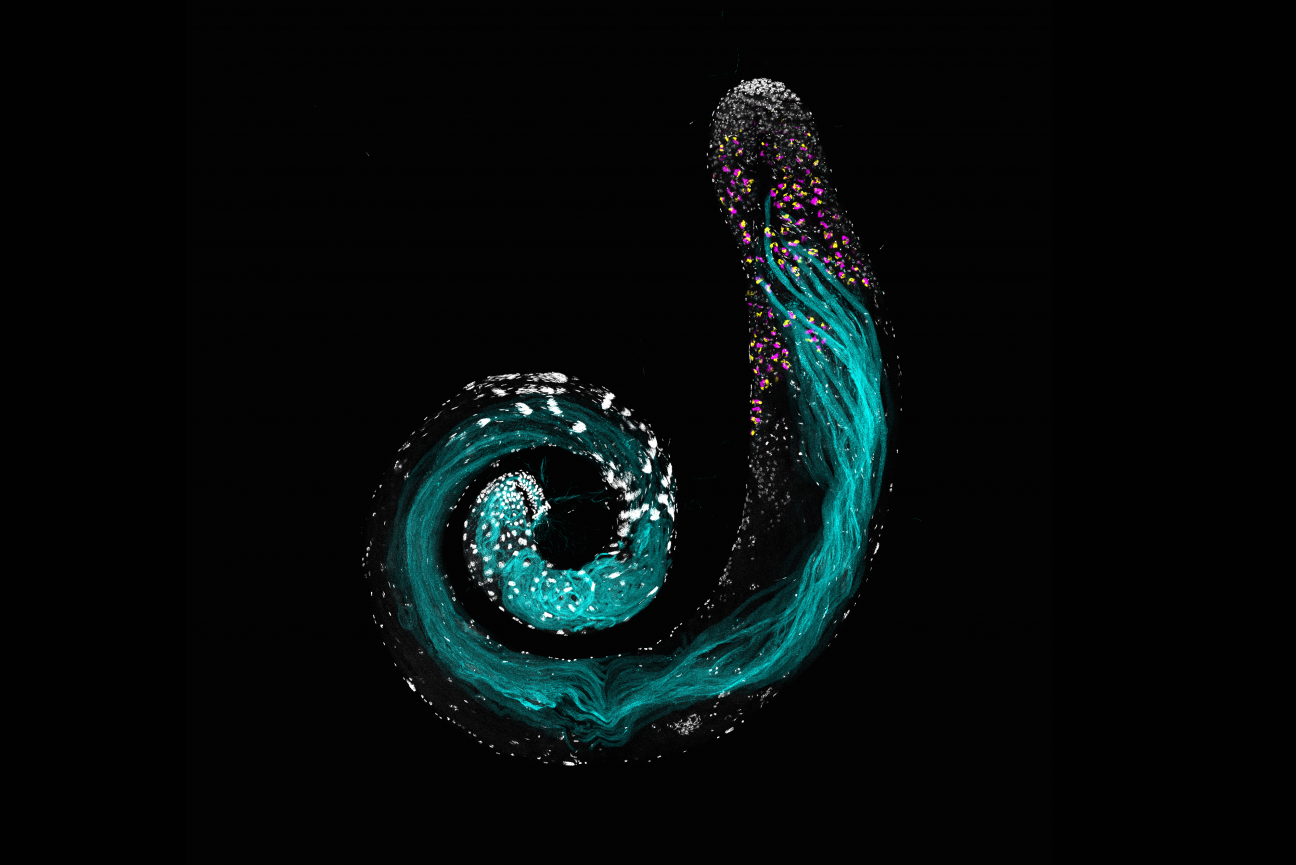
The Drosophila testis. DNA is in white. The section in white at the top of the image is where the germline stem cells are found. Bundles of white in the center of the spiral are the nuclei of mature sperm, which have differentiated from germ stem cells. White along the spiral shows different cell types along the path of germ cell differentiation. Sperm tails are in cyan. RNAs specific to an early stage of sperm development are in magenta and yellow.
Jaclyn Fingerhut/ Whitehead Institute
Fruit flies may seem like a nuisance when they suddenly start multiplying in the kitchen trash, but the contributions they have made to science are multitudinous. With the tools to manipulate their genomes, their short generation time, large numbers, and easily observable development, fruit flies make for an excellent model organism, and the success of Drosophila research over the decades bears that out. They have been instrumental in shaping our understanding of genetics, development, health and disease, and more. The many flies inside of Whitehead Institute will help answer important questions about how life begets new life, and how new life develops during its early stages.

A loose fly in the lab rests on a centrifuge.
Jaclyn Fingerhut/ Whitehead Institute
Special thanks to Ruth Lehmann, Yukiko Yamashita, Jaclyn Fingerhut, Mariyah Saiduddin, and Ruoyu Chen.
Cover video by Jaclyn Fingerhut.
Citations:
[1] Pandey, Udai Bhan, and Charles D Nichols. “Human disease models in Drosophila melanogaster and the role of the fly in therapeutic drug discovery.” Pharmacological reviews vol. 63,2 (2011): 411-36. doi:10.1124/pr.110.003293
[2] “Thomas H. Morgan – Biographical.” NobelPrize.org. Nobel Prize Outreach AB 2021. Sun. 1 Aug 2021. https://www.nobelprize.org/prizes/medicine/1933/morgan/biographical/
[3] “The Nobel Prize in Physiology or Medicine 1995.” NobelPrize.org. Nobel Prize Outreach AB 2021. Sun. 1 Aug 2021. https://www.nobelprize.org/prizes/medicine/1995/summary/
[4] Pitnick, Scott, et al. “How Long Is a Giant Sperm?” Nature, vol. 375, no. 6527, 1995, pp. 109–109., doi:10.1038/375109a0.
Contact
Communications and Public Affairs
Phone: 617-452-4630
Email: newsroom@wi.mit.edu