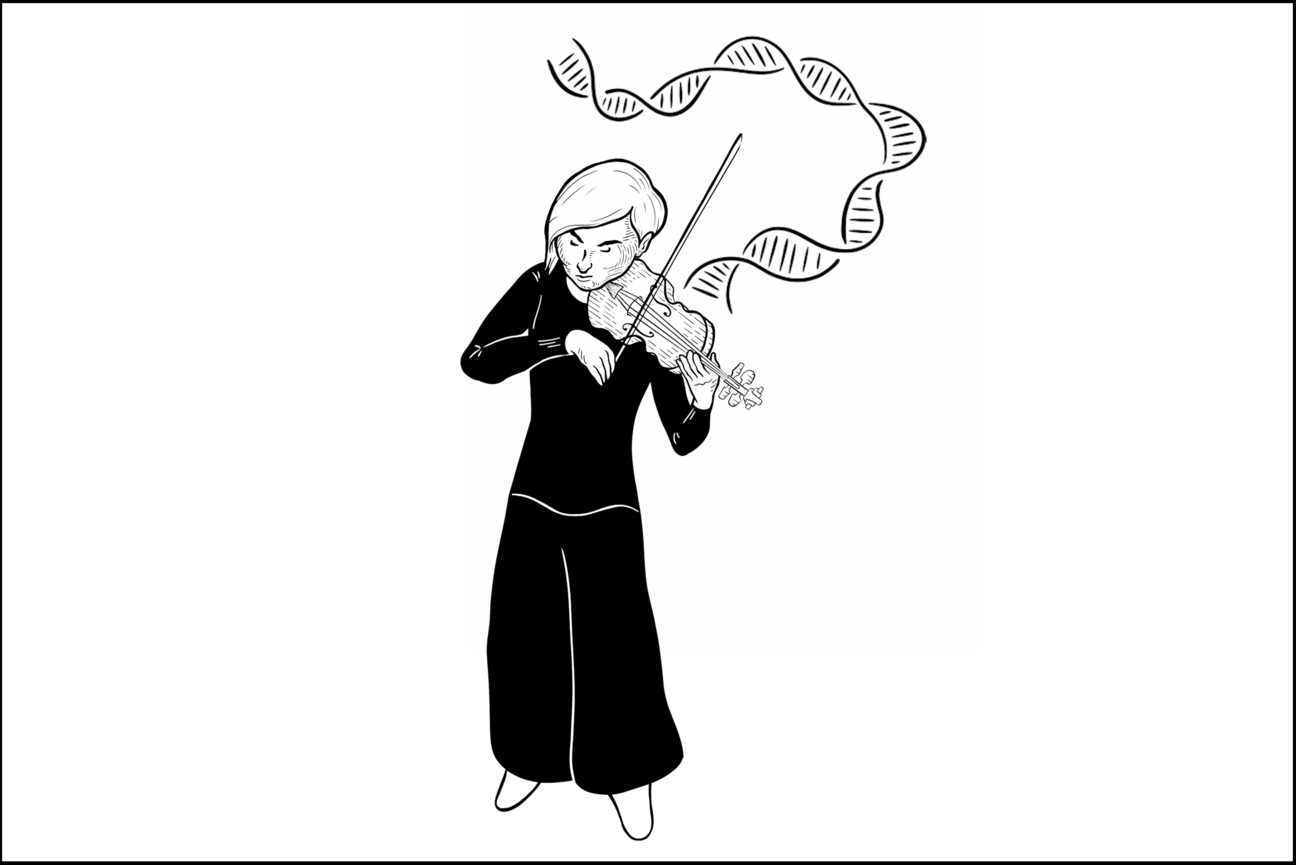
Devin Powell/Whitehead Institute
A reimagined glimpse of cellular symphony
Cells are considered the most basic units of life, but within each cell lies a hidden, vast library of instructions called genes. Like instruments in an orchestra, thousands of genes work together, with the help of transcription factors and signaling pathways, to guide the production of proteins that keep the cell, and the symphony of life, functioning smoothly.
Historically, researchers have lacked the tools to fully decode the orchestral score for this symphony and connect the extremes of scale — the precise roles of individual genes and their broader interactions within the system.
Now, scientists at Whitehead Institute are bridging this gap with advanced techniques that enable investigation of complex biological questions at both the micro and macro level. Their work, together, is leading to a much richer understanding of the inner workings of cells and the mechanisms underlying diseases.
Fine tuning the instrument: genetics
Just as a musician might tighten the strings of their violin to alter its sound, genes can undergo epigenetic changes — such as the addition of chemical tags that modify their expression — without altering the genetic code itself, influencing how a gene functions within the cellular orchestra.
The lab of Whitehead Institute Member Rudolf Jaenisch is interested in epigenetic mechanisms that control gene expression in neurodevelopmental disorders like Rett and Fragile X syndrome. Most recently, work from the Jaenisch Lab focused on the MECP2 gene, which is implicated in Rett syndrome.
Researchers have known that the MECP2 protein regulates gene expression by binding to DNA and influencing the expression level of numerous genes essential for neuron health. However, developing therapies for Rett syndrome necessitates a deeper understanding of MECP2 and its diverse functions in the brain.
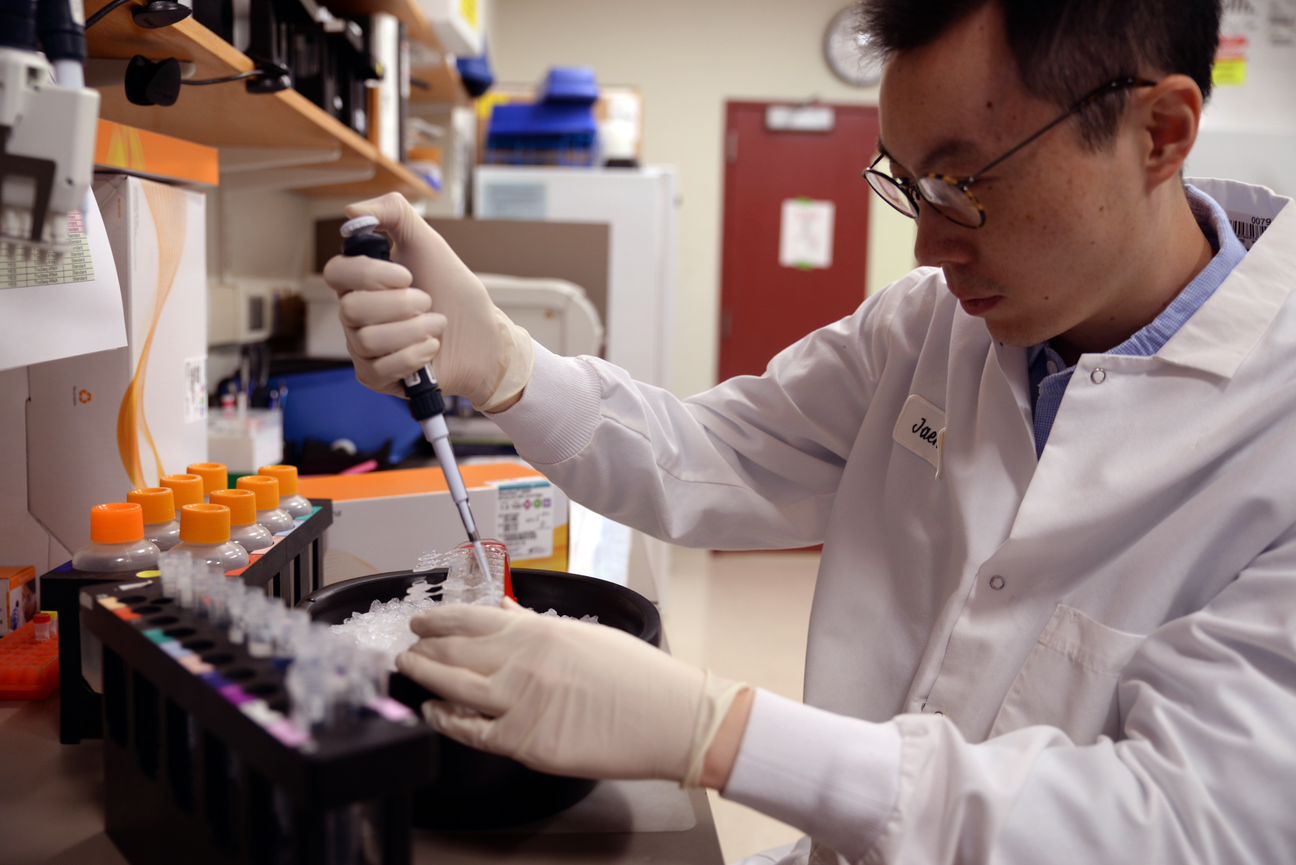
To understand MECP2’s full role in Rett Syndrome, postdoctoral fellow Yi Liu uses an advanced genetic technique called CUT&Tag that will help his team precisely identify protein-DNA interactions.
Madeleine Turner/Whitehead Institute
To understand the gene’s full role, the team turned to an advanced genetic technique called CUT&Tag. Using this method, the researchers were able to identify protein-DNA interactions with high sensitivity and specificity, helping create a comprehensive map of MECP2’s binding sites in both healthy and mutation-carrying human neurons.
The researchers’ unique approach to studying MECP2 has unveiled new insights into the complex molecular mechanisms involved in Rett syndrome and how they might one day be addressed in order to ameliorate the disease.
Orchestrating gene function across cells: genomics
Yet, with millions of cells in the human body and a lack of large-scale cell imaging tools, biologists face limitations in visualizing gene function across numerous cells simultaneously. It’s almost like trying to pick up the sound of each individual instrument in an orchestra.
To address this long-standing problem in cell biology, Whitehead Institute Member Iain Cheeseman and collaborators have integrated two complementary approaches — large-scale CRISPR/Cas9 screens and cell imaging — enabling scientists to probe the activity of thousands of genes across time and space in human cells.
“For my entire career, I’ve wanted to see what happens in cells when the function of an essential gene is eliminated,” says Cheeseman. “Now, we can do that, not just for one gene but for every single gene that matters for a human cell dividing in a dish, and it’s enormously powerful. The resource we’ve created will benefit not just our own lab but labs around the world.”
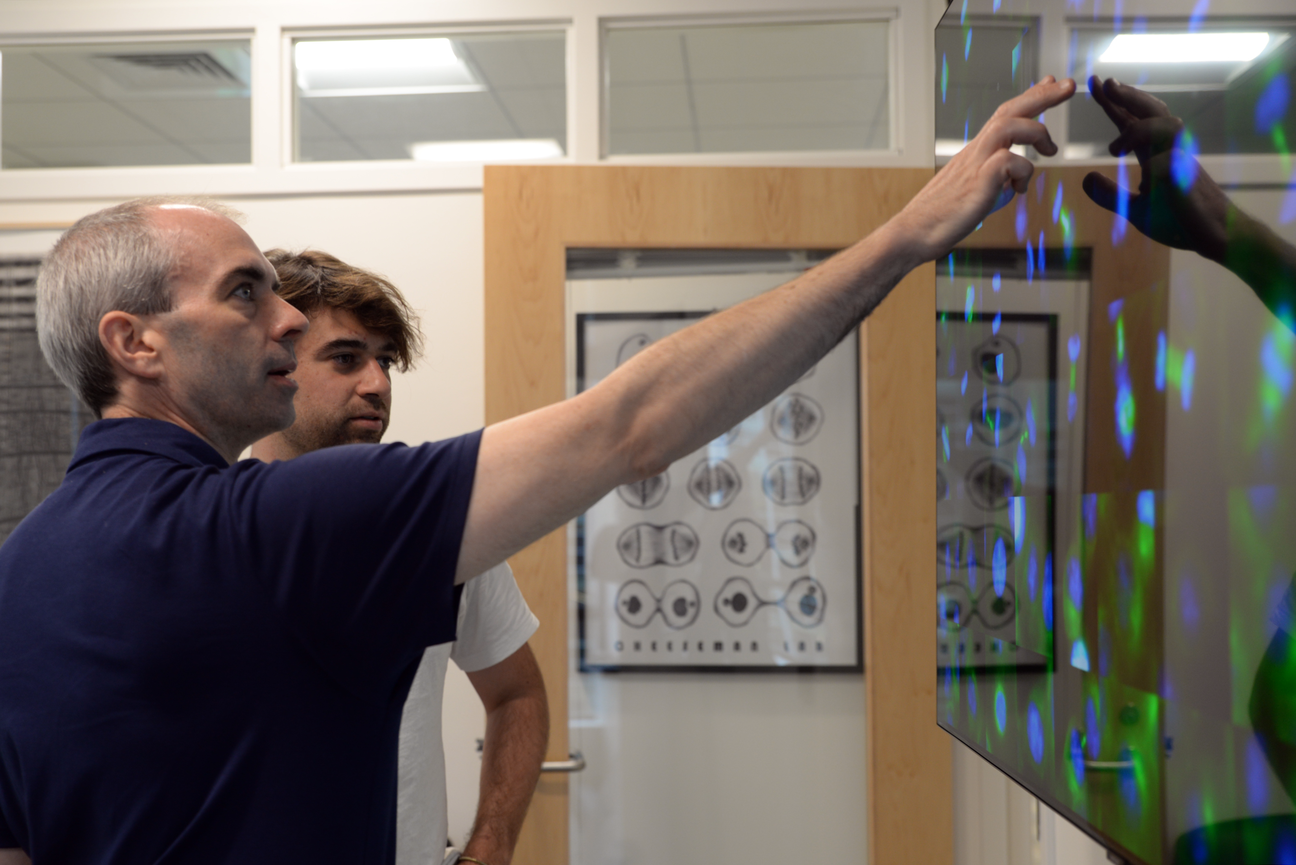
Whitehead Institute Member Iain Cheeseman and graduate student Matteo Di Bernardo look at images from a large-scale optical screen.
Madeleine Turner/Whitehead Institute
In fact, the lab of Whitehead Institute Member Sebastian Lourido is taking a similar approach to studying genes in the parasite Toxoplasma gondii. With the help of CRISPR gene-editing technology, the group has been able to systematically turn off each of the 8000 genes in the parasite’s genome to observe how these knockouts affect its growth and the infection process in a mouse model.
“You can imagine that a parasite infecting a single cell is going to be quite different from how it behaves in an animal,” says Christopher Giuliano, a graduate student in the Lourido Lab. “During a real infection, there’s factors like metabolism and immunity that are incredibly hard to capture in cell culture.”
Prior to this study, the researchers had conducted smaller-scale traditional CRISPR screens in mice by injecting mutant parasites and then retrieving them in order to analyze the effects of genetic disruptions on the parasites’ growth. This time, they’ve added a “barcode” to their mutant parasites, allowing them to do genome-wide screens that help track the fitness of the parasites more precisely within the host organism.

Graduate student Christopher Giuliano cultures T. gondii in human fibroblasts.
Madeleine Turner/Whitehead Institute
Conducting the cell: multi-omics
Carefully integrating the sounds of different instruments in an orchestra is crucial to developing a symphony. Researchers at Whitehead Institute are taking a similar approach to understanding biological processes. They’re piecing together different layers of data using an approach called multi-omics, which combines information about genes, proteins, and other molecules in a living organism, to gain a deeper understanding of how the organism functions.
For example, by combining the CRISPR/Cas9 gene editing technology with single-cell RNA sequencing — in an approach known as Perturb-Seq — the Weissman Lab has created the first comprehensive functional map of genes expressed in human cells.
The CRISPR/Cas9 genome editing technology helps introduce genetic changes into cells, while single-cell RNA sequencing captures the resulting RNA expressions. Together, these genomic tools help decode the complex effects of genetic perturbations by analyzing the pattern of gene expression in cells over time.
Now the Weissman Lab is eager to use Perturb-Seq in a mouse model to investigate organ pathophysiology. “Part of the excitement of the project is that it goes all the way from basic CRISPR engineering to thinking about genuine human patient liver pathophysiology,” says Reuben Saunders, a graduate student in the Weissman Lab. “I think it’s the closest I’ve ever gone across the entire spectrum.”
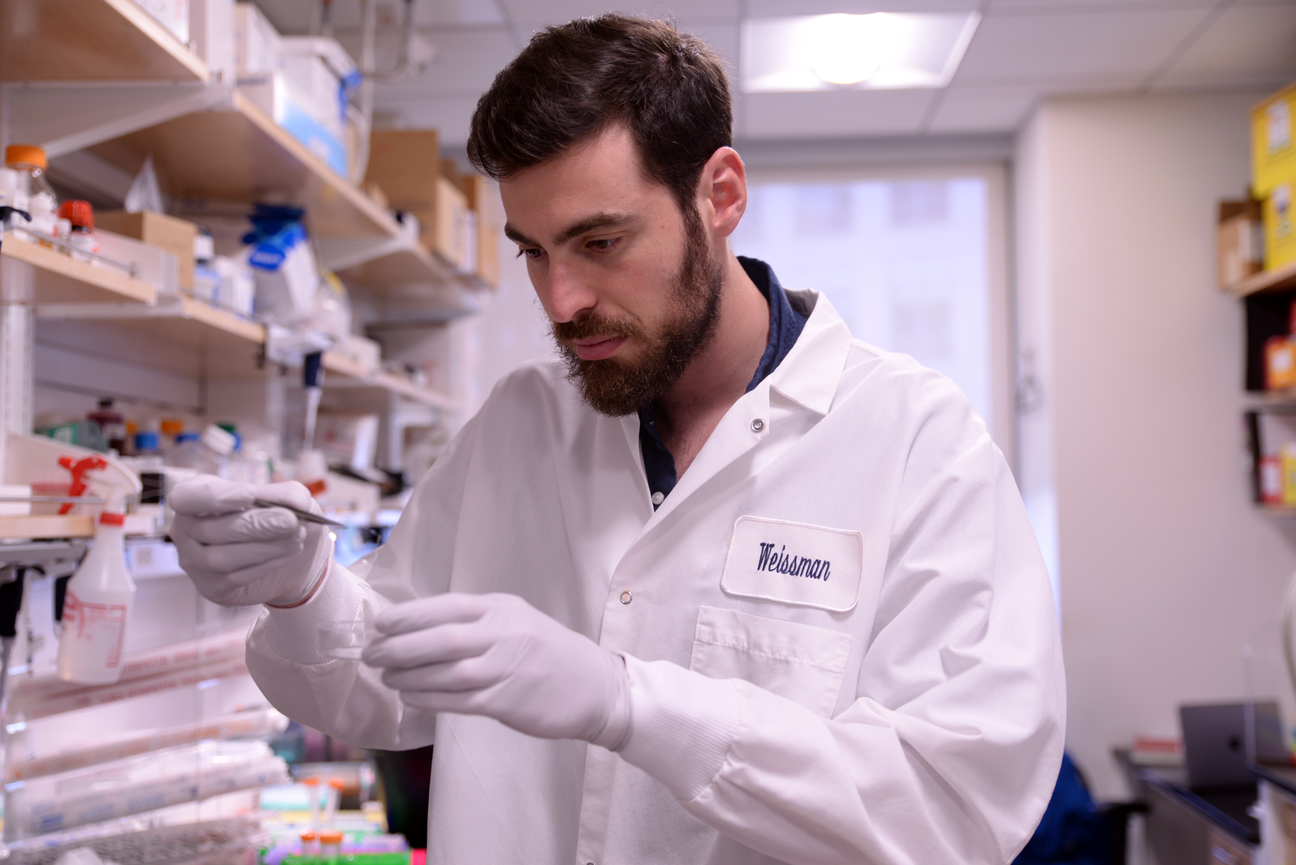
Graduate student Reuben Saunders uses Perturb-Seq in mouse organs to identify which genes can be turned on or off to influence normal biological processes.
Madeleine Turner/Whitehead Institute
But Perturb-Seq isn’t the only instance of the Weissman group integrating multiple genomic approaches in their investigations. In a recent series of studies, the researchers developed and deployed a lineage tracing method that has allowed them to follow how tumors initiate, grow and metastasize with unprecedented precision.
Orchestral score of a harmonious symphony: computation
These projects are just some examples, among many, of how Whitehead Institute researchers are generating multi-dimensional data of unprecedented complexity and volume. But these vast datasets — a treasure trove of hidden patterns and correlations — can often elude the human brain.
Whitehead Fellow Allison Hamilos is applying machine learning to these complex datasets in order to advance her research in neuroscience. Her lab investigates brain circuits responsible for regulating reward and movement to understand the mechanisms that go awry in conditions like Parkinson’s, schizophrenia, and bipolar disorder.
With the help of machine learning vision models that monitor and analyze body movements as mice engage in complex behaviors, alongside advanced tools like electrophysiology and optogenetics that track and manipulate the activity of specific neural cell populations, Hamilos and her team can precisely capture differences between healthy and disease-affected neural circuits.
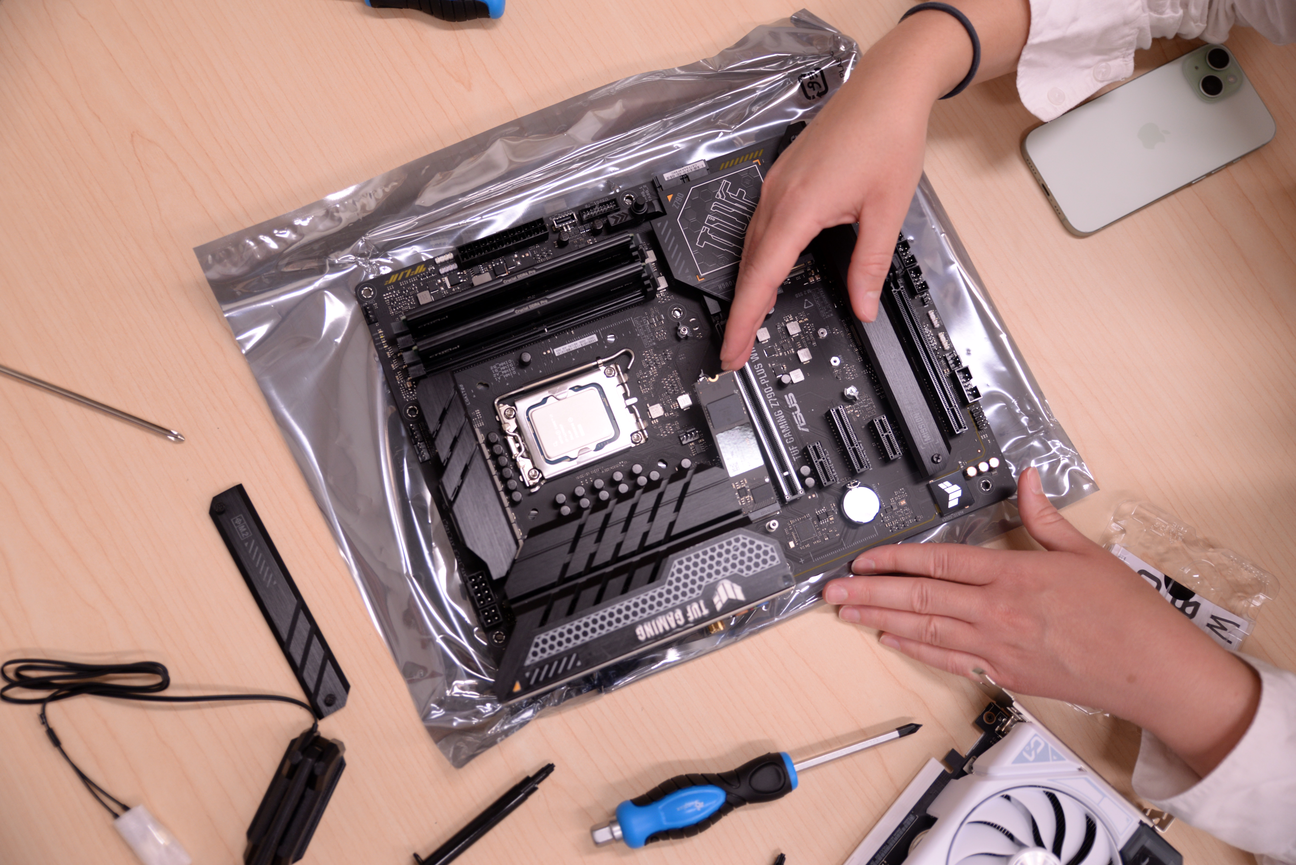
Valhalla Fellow Allison Hamilos and her lab build their own computers and electronics hardware to decode the language of cells in the brain with techniques from artificial intelligence and machine learning.
Madeleine Turner/Whitehead Institute
Whitehead Institute Member Richard Young shares Hamilos’ enthusiasm in leveraging AI to make meaningful predictions that can advance our understanding of biological systems.
His research on condensates — tiny, membrane-less organelles within cells where biomolecules essential for gene function concentrate — has upended the traditional view of cell biology and gene regulation. Building upon the group’s previous insights into how condensates affect drug behavior, the Young Lab is now refining a machine learning model to predict where a drug will concentrate within the cell depending on its chemical properties.
“Our work suggests that if you want to develop a very efficacious drug, then you should know where the target of the drug is in the cell with respect to these compartments,” says Young. “This would inform researchers and companies of the best way to develop a drug so that it is optimally concentrated near its target.”
To explore other stories in this collection, highlighting Whitehead Institute researchers’ work across scales of size and time, click here.
Topics
Contact
Communications and Public Affairs
Phone: 617-452-4630
Email: newsroom@wi.mit.edu