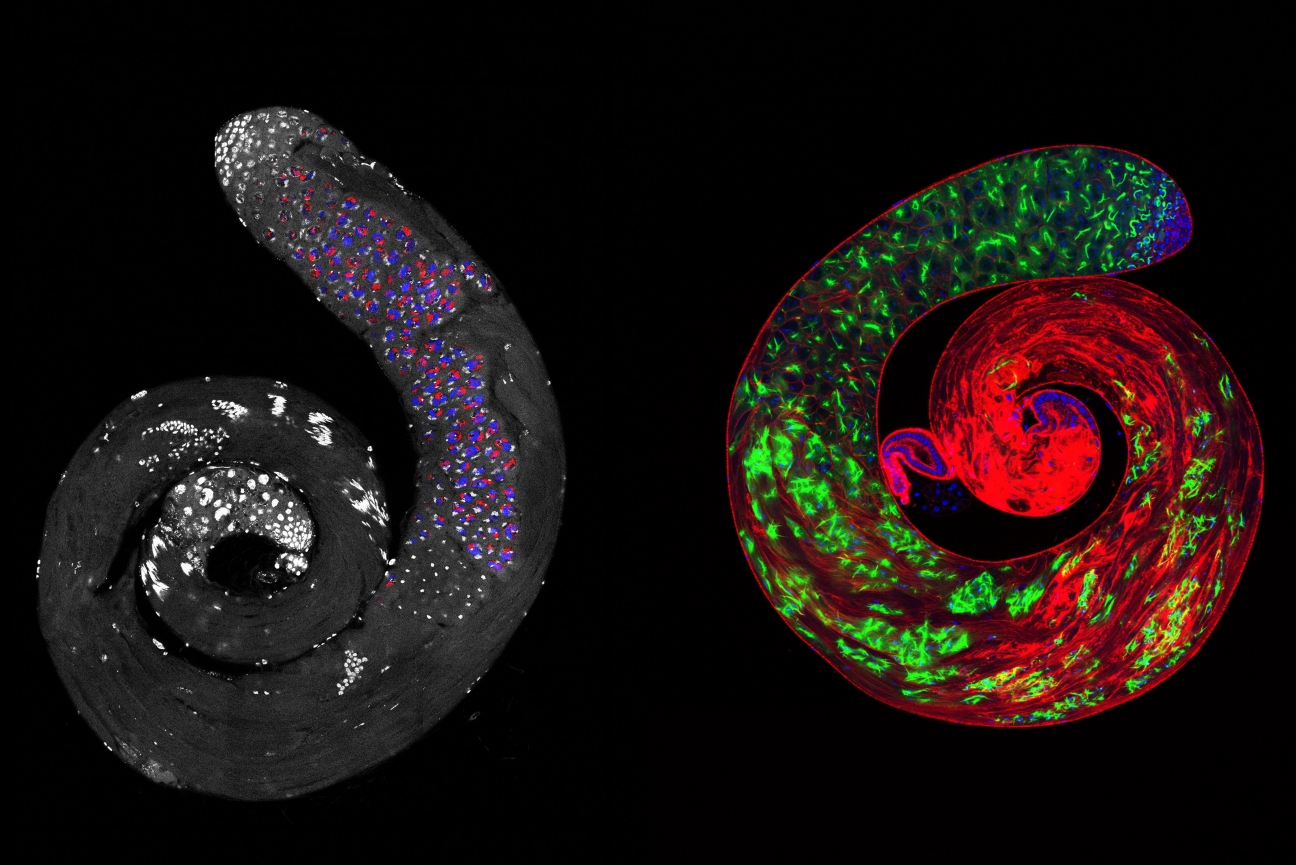
Drosophila testes with stain. Whitehead Member Yukiko Yamashita uses Drosophila as a model organism to study the behavior of germ cells.
Yukiko Yamashita
The immortality of germ cells
This article is part of our series on rejuvenation research at Whitehead Institute. Click here to view the entire collection.
Germ cells, the reproductive cells that eventually become eggs and sperm, are set aside early in embryonic development, when the embryo is just a hollow ball of cells called the gastrula. This means that the cells transferring genetic information to the next generation are already with us at birth.
There are many questions that researchers are still trying to understand about the immortality of the germline. "Not many people truly appreciate the crazy mystery of how germ cells have lasted 1.5 billion years," Whitehead Institute Member Yukiko Yamashita says. Researchers at Whitehead Institute, including Yamashita and Whitehead Institute Director Ruth Lehmann, study the many aspects of germ cells that set them apart from other cell types. They ask questions about how germ cells migrate across the embryo to meet developing gonads, and how germ cells ultimately give rise to eggs and sperm.
A central question in both Yamashita and Lehmann's work is how germ cells use a unique regulatory system to maintain the potential to divide into any cell type, without actually doing so for decades on end. When germ cells produce progeny, the program of aging is reset, and the cells in the progeny begin afresh. This feature is in sharp contrast to most of the non-reproductive cells in the body, which differentiate into specialized cell types, age, and eventually die.
Credit: Jennifer Cook-Chrysos
Learning how germ cells maintain their DNA in order to produce fit eggs and sperm, generation after generation, may lead to insights into how other cells throughout the body age. By better understanding how germ cells are maintained, it might one day be possible to slow or reverse aging processes in other cells.
Creating the germ line
Early in embryonic development, the germline is set aside from the cells that make up the rest of the body, which are called somatic cells. The cells set aside are the predecessors of the mature germ cells that can make eggs or sperm, and these immature cells are called primordial germ cells.
Lehmann has uncovered many aspects of how germ cell fate is assigned by studying female fruit flies. As a graduate student, Lehmann identified and characterized genes essential to the proper formation of the germline. Germ cell precursors form at one tip—the back end—of an early fly embryo. Lehmann found that a gene she named Oskar plays a key role in assembling the necessary ingredients for germ cells in this location. Oskar helps to seed germ granules, droplet-like structures unique to the germline that form as RNA and proteins assemble together. Germ granules regulate the translation of RNA into protein, thereby controlling gene expression, and helping germ cells to form and develop properly.
Primordial germ cells form before the embryo begins making a reproductive tract. As the gonads—ovaries or testes—develop, the primordial germ cells must migrate through the embryo to reach them. Whitehead Institute researchers have helped discover how the cells are guided on this journey and mature into cells capable of making eggs or sperm. Lehmann’s lab has found factors involved in this migration. For example, they identified the signaling molecule that provides a trail of breadcrumbs for germ cell precursors to follow to their destination.
In recent work, Lehmann’s lab figured out how the fly’s body signals germ cell precursors to differentiate into mature germ cells in the right time and place—in the reproductive tract, once the ovaries are ready to host eggs—as well as what signals keep the precursors from maturing too early. Led by former Lehmann lab postdoc Torsten Banisch, the researchers identified swarm cells, whose function was previously unknown, as a critical relay that transmits cues to germ cell precursors that signal them to mature. If any of these processes goes awry—if the cells migrate to the wrong location, mature at the wrong time, or fail to receive the signal to mature—the fly is likely to lose its germline and become infertile.
Maintaining a healthy germline
We commonly think of cell division as one cell splitting into two identical copies. However, cells can divide asymmetrically, creating two daughter cells that are not identical to each other. Yamashita studies germ cells in male fruit flies and has found that dividing germ cells pack one of the resulting cells full of only the best components at the expense of the other cell. For example, Yamashita found that one of the resulting cells will receive the other’s copies of certain genetic sequences that otherwise can be lost with age over the course of many cell divisions. The lab also found that chromosome pairs are non-randomly sorted into dividing germ cells, based on which has more copies of the genetic sequences that need to be maintained through generations.Their finding shows that this is one way the germline prevents loss of important genetic sequences with aging. The cell that gets all of the best materials continues dividing, keeping the germline healthy and fit for generations. Stem cells outside of the germline also divide asymmetrically, in order to allow some of their descendants to mature while others maintain the stem cell pool. Yamashita’s work with germ cells sheds light on this broader biology, delving into various mechanisms that enable asymmetric cell division.
For other cell types, sacrificing half of their cells in the way that the germline does to maintain its health would be too resource intensive to afford—every cell is needed to keep the body going—and so over many divisions our body’s cells degrade as we age. Germ cells are a small cell population that can afford to let only the cream of the crop survive—in fact, they need to be choosy in order for their descendants to keep dividing long after the rest of the body’s cells die. Discovering the processes like this that germ cells use to maintain their immortality could also provide insights into other immortal cell types, like cancers.
Lehmann has also uncovered some of the ways in which germ cells are able to maintain themselves throughout the generations. Her research illuminated how germ cells in flies protect their genomes from transposable elements or “jumping genes.” These are DNA sequences that can move to new positions in the genome, which can lead to mutations or change in genome size. Germ cells must be protected from such changes to their genomes, as these will either be passed down to offspring or could degrade the germ line to the point of infertility, putting an end to the cells’ immortality. Lehmann has discovered how germ cells prevent such changes.
Lehmann also studies inheritance of non-DNA components in the maternal germline. All germ cells must carefully maintain their DNA so it can be passed down to the next generation. The maternal germ line’s job does not end there, however; egg cells must pass on not only the mother’s DNA, but nutrients, starter organelles, and cellular components—such as RNA—that the earliest stages of the embryo rely on to function before they can ramp up production of their own components. One organelle of particular interest to Lehmann is the mitochondria, a structure that provides energy for cells. Mitochondria have their own genome, unique to them, and mutations to mitochondrial DNA can cause serious diseases. Lehmann’s lab studies how the maternal germline prevents passing on dangerous mutations in the mitochondrial genome.
As Yamashita and Lehmann continue studying the complex ways that germ cells preserve their immortality, they hope to discover insights into cellular aging and rejuvenation.
Contact
Communications and Public Affairs
Phone: 617-452-4630
Email: newsroom@wi.mit.edu