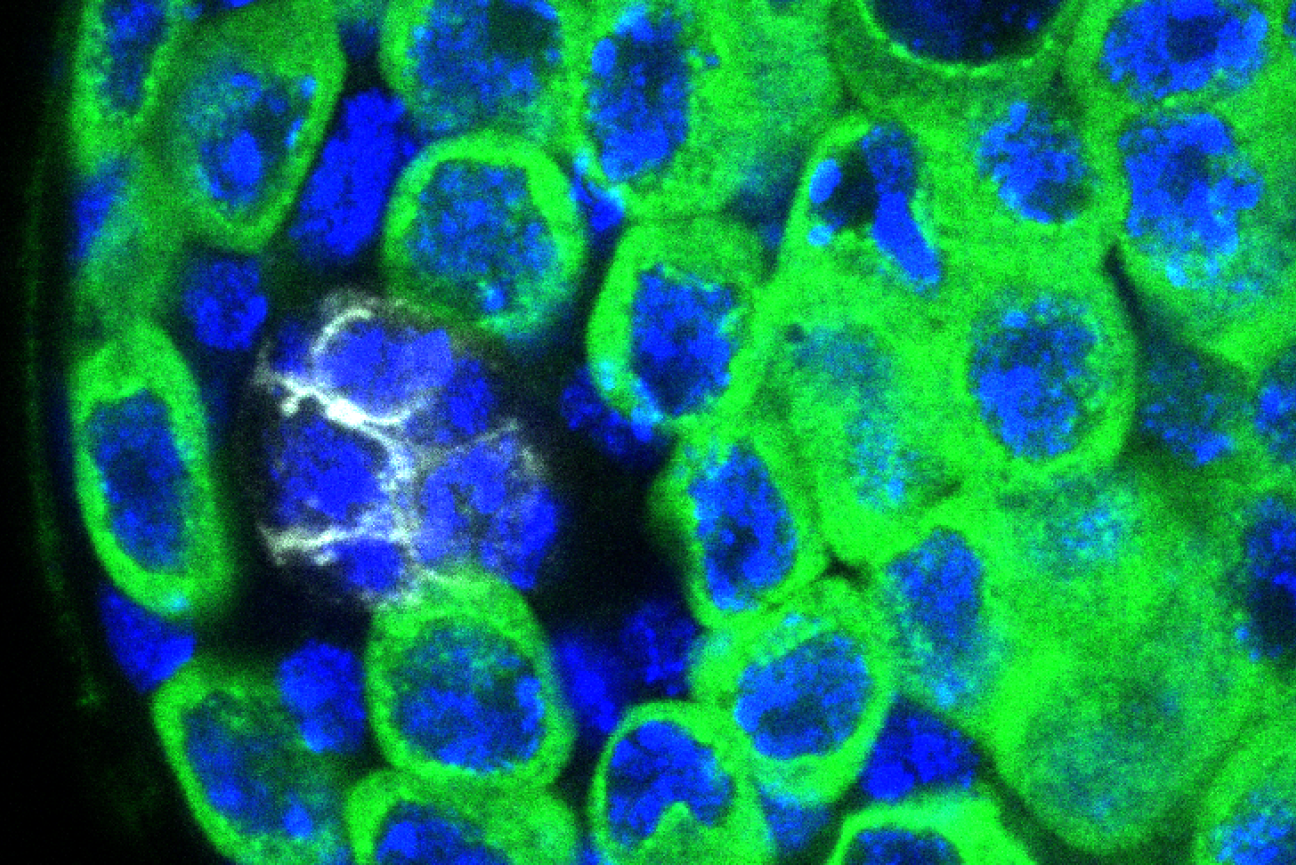
Fruit fly germline stem cells, the cells that make sperm or eggs.
Jonathan Nelson/ Whitehead Institute
Help from an unexpected corner: a “genetic parasite” protects fertility
This story is part of our series, "Unsung cellular heroes." To read more stories in the series, click here.
Ribosomal DNA (rDNA) sequences are essential parts of species' genomes. The highly repetitive nature of these DNA sequences makes them susceptible to being deleted over time by the cell’s division or repair mechanisms—and if too many are deleted, cells can die. If too much rDNA gets deleted in germ cells, the cells that become eggs and sperm, then individuals may become infertile and their lineages may go extinct. Researchers have suspected that something helps to keep our rDNA intact over the generations—keeping humans and other species fertile—but they did not know what it was. Recent research from Whitehead Institute Member Yukiko Yamashita and postdoc Jonathan Nelson has revealed rDNA’s unlikely protector: a retrotransposon, a genetic element that had been thought of as a genetic parasite because it seems to exist only to replicate itself. Their research explains how this so-called parasite actually plays an essential role in maintaining rDNA and so preserving fertility.
The puzzle of why rDNA does not disappear
rDNA generates the RNA subunits of ribosomes, the cellular machines that make proteins, the cells’ essential workers, by following the instructions encoded in genes. Our cells require many ribosomes to make all of the proteins they need to function, so our genome is full of repeated copies of the rDNA sequence for making ribosome parts. The problem with this kind of repetitive DNA is that it’s easy for the cell to accidentally remove some of the identical repeats when the genome is being duplicated during cell division. Over time, as cells go through multiple divisions, the number of repeats would be expected to get smaller and smaller. This problem would be particularly noticeable in the cells of aging individuals and in germ cells, the only cells that get passed from one generation to the next. If nothing were helping rDNA to recover its missing repeats, then each new generation would start out with fewer repeats than the last, until a generation did not have enough repeats left to make viable germ cells—and so that population would die out.
Yamashita, who is also a professor of biology at the Massachusetts Institute of Technology and an Investigator with the Howard Hughes Medical Institute, studies germ cell immortality in male fruit flies (Drosophila melanogaster), or how germ cells can keep making healthy sperm and eggs throughout many generations of individuals. Every other type of cell dies along with the body in which it is born, and so the genomes of these cells can accrue some damage over time—such as losing repeats in their rDNA—without much consequence. However, errors in the germ cell genome can accumulate over the generations, so germ cells must be especially careful to maintain their rDNA in order to preserve their immortality. When germ cells lose too many rDNA repeats, they are able to replace them with new repeats, but no one has known how they were able to do this. Nelson and Yamashita set out to find the answer.
“Ribosomal DNA is repetitive and so is bound to be lost, and the logical consequence is that we should all lose the rDNA in our germ cells and the future generations would be totally gone,” Yamashita says. “So how come that hasn't happened yet? This is the kind of question that’s so big you don’t even see it at first—you take it for granted that something is maintaining rDNA—but once we saw that the question was there, we needed to find the answer.”
Retrotransposons: not so selfish after all
What the researchers discovered is that rDNA is restored with the help of a retrotransposon, R2. Retrotransposons are genetic sequences whose primary function is to replicate themselves, even at the expense of the rest of the genome. They have been called genetic parasites but their behavior is most similar to that of a virus, which manipulates cells into making copies of itself. The way a retrotransposon makes more copies of itself is by reversing the usual process of gene expression. When the DNA coding for a retrotransposon is read into RNA, that RNA can be read back into DNA. The retrotransposon then slices open the cell’s genome and inserts its new DNA, adding another copy of itself to the genome. This process not only balloons the size of a species’ genome over generations—nearly half of the human genome consists of transposable elements—but it can also cause damage to an individual cell. When a retrotransposon cuts into the genome, especially if it then inserts itself into the middle of a necessary DNA sequence, it can make genes unusable.
Sidebar
Retrotransposons’ gene editing activity sometimes introduces other sequences into the genome. For example, Whitehead Institute Member Rudolf Jaenisch found that the retrotransposon LINE1 enables the SARS-CoV-2 virus responsible for Covid-19 to, on rare occasions, insert part of its genome into the human genome when it has infected human cells. Retrotransposons’ gene editing has even been indirectly helpful at times, as the side effects of their DNA shuffling can lead to increases in human genetic diversity.
However, Nelson and Yamashita found that the retrotransposon R2, which typically copies and inserts itself into fruit fly rDNA, can also help cells much more directly. In a dividing cell, there are two copies of each chromosome—one to go in each of the new daughter cells. R2 slices open both copies of the chromosome containing rDNA. When the cell tries to repair these breaks, the repetitive nature of the rDNA can essentially make it lose its place, so it stitches a stretch of rDNA repeats from one copy of the chromosome into the other copy of the chromosome instead. This means that one of the daughter cells will end up with more repeats in its rDNA than the original cell had, while the other daughter cell will have fewer repeats. The germ cells can then protect their immortality by making sure that the cell with more repeats in its rDNA is the one used to keep the germline going.
Work from Yamashita’s lab, published in 2022, identified how germ cells make this selection. Germ cells divide asymmetrically, so one of the new daughter cells remains a germline stem cell, continuing to make more germ cells, and the other daughter cell differentiates or begins down the path of making sperm. Yamashita lab postdoc George Watase and Yamashita discovered a gene, which they named Indra, that creates a protein that attaches to the copy of the chromosome containing more rDNA repeats. This protein marks the daughter cell containing that chromosome to remain a stem cell, while the other daughter cell goes on to make sperm.
Germ cells can combine these approaches, taking rDNA repeats from one chromosome to give to another and then earmarking the cell with more repeats, to constantly replenish the germline’s level of rDNA. This ensures that the number of rDNA repeats never gets too low across the population of germ cells, preserving the lineage of the cells and the individuals who carry them.
Nelson and Yamashita’s work shows that R2 is not merely a selfish parasite, but instead plays a pivotal role in this process of germline rDNA rejuvenation. However, as a retrotransposon, R2 is also capable of causing damage. Nelson found that germ cells keep R2 inactive except in cases where the number of repeats in rDNA is too low. In this way, the cells may maximize the benefits of R2 and minimize its dangers, by only accepting the risk of damage when needed. This may allow the cell and retrotransposon to have a mutually beneficial relationship. Yamashita and Nelson speculate that other transposable elements may likewise provide unknown benefits to the cell.
“A lot of transposable elements are thought of as existing because their ability to replicate in the genome is better than the ability of the host to defend itself from that replication,” Nelson says. “These elements make up large regions of the genome that we think of as non-functional, but what if the reason why there are so many of them is because they contribute some function that we just don't understand yet?”
Sidebar image credit: Conor Gearin/ Whitehead Institute
Citations:
George J. Watase, Jonathan O. Nelson, Yukiko M. Yamashita. “Nonrandom sister chromatid segregation mediates rDNA copy number maintenance in Drosophila.” Science Advances, July 27, 2022. https://www.science.org/doi/10.1126/sciadv.abo4443
Jonathan O. Nelson, Alyssa Slicko, Yukiko M. Yamashita. "The retrotransposon R2 maintains Drosophila ribosomal DNA repeats." PNAS, May 30, 2023. https://doi.org/10.1073/pnas.2221613120
Liguo Zhang, Alexsia Richards, M. Inmaculada Barrasa, Stephen H. Hughes, Richard A. Young, and Rudolf Jaenisch. "Reverse-transcribed SARS-CoV-2 RNA can integrate into the genome of cultured human cells and can be expressed in patient-derived tissues." PNAS, May 6, 2020.
Liguo Zhang, Punam Bisht, Anthony Flamier, M. Inmaculada Barrasa, Max Friesen, Alexsia Richards, Stephen H. Hughes, and Rudolf Jaenisch. 2023. "LINE1-Mediated Reverse Transcription and Genomic Integration of SARS-CoV-2 mRNA Detected in Virus-Infected but Not in Viral mRNA-Transfected Cells" Viruses 15, no. 3: 629. https://doi.org/10.3390/v15030629
Contact
Communications and Public Affairs
Phone: 617-452-4630
Email: newsroom@wi.mit.edu