
Steven Lee/Whitehead Institute
When the blueprint shifts: research at Whitehead Institute into developmental disorders, diseases, and differences
This article is part of our Building a Body story collection. Read the entire collection here.
A technician presses an ultrasound wand into the stomach of a pregnant person. On the screen in front of them, a small oblong shape appears: a healthy embryo, busy growing and developing. The technician measures the embryo, and later the doctor tells the person the exact age of the embryo. This is possible because our bodies develop in a well-known, well-timed sequence of events. Researchers have learned exactly when an embryo will start to grow lungs, a heart, a brain–when its nose will take shape on its growing head. However, as the embryo grows, factors like its genes or its environment can alter its growth and, sometimes, cause its development to diverge from the familiar pattern. These differences can have lifelong effects.
Whitehead Institute researchers study how differences arise in development in order to better understand our bodies, and to learn how we might prevent or treat cases in which development goes awry. Whitehead Institute scientists have discovered important biology underlying developmental disorders such as Rett syndrome, microcephaly, and Fragile X syndrome, and have identified how situations that arise during development can lead to a higher risk of different cancers down the road. Whitehead Institute researchers are also exploring how having different combinations of sex chromosomes, the X and Y chromosomes that correspond to female or male biological sex, can shape human health and disease from early development onwards. These research projects continue to provide important insights into human biology, and they are paving the way for future treatments to address developmental disorders and diseases.
Building the brain
Whitehead Institute Founding Member Rudolf Jaenisch’s lab uses stem cell technology to study tissues throughout the body, but much of his lab’s work has focused on the development of one crucial organ: the brain. One of the major challenges that researchers in the Jaenisch lab must overcome is how to accurately model what happens inside a developing brain—or any other part of the body—in the lab. Researchers cannot study a developing fetus’ brain cells directly, and animal models do not always provide close enough matches to be a good stand-in. Instead, the lab uses stem cell models. They learn the right cocktail of signals to turn stem cells into whatever cell type they are interested in studying so they can experiment with those cell types directly. The Jaenisch lab has been the first to create several stem-cell derived cell types, including microglia, the brain’s immune cells. The lab is also leading the way in making stem cell models as close to the biology of a living human body as possible, in order to make the models as accurate as possible.
“The key problem of stem cell biology is that it is difficult, if not impossible, to turn stem cells into functional and mature cells that resemble primary cells directly taken from a patient,” Jaenisch says. “That’s a big problem that our lab is focused on addressing.”
Their strategies include coming up with new recipes to make stem-cell derived cell types better matches for the body’s cells, as well as growing cells in “organoids,” three-dimensional structures that can imitate some of the body’s complexity, such as interactions between cells, rather than simply studying cells in two dimensions in a dish.
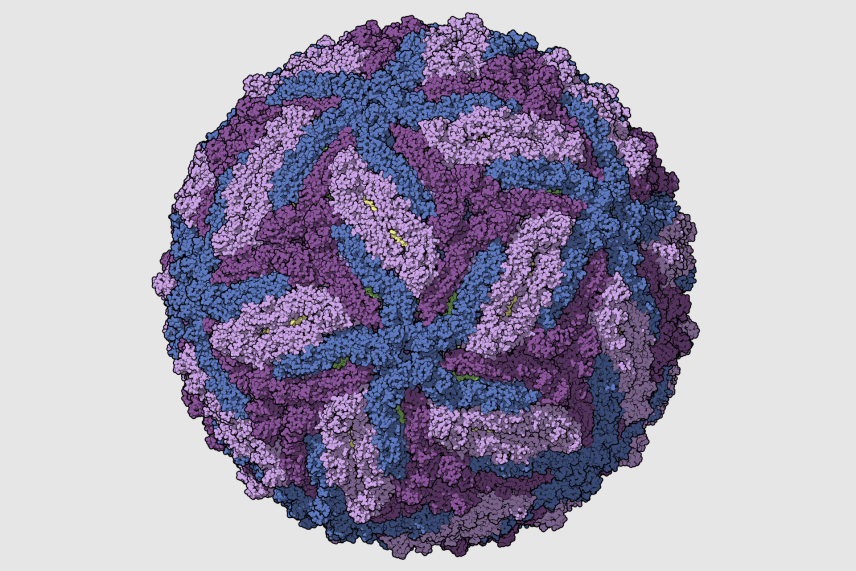
Zika virus capsid, colored per chains.
Sirohi, D., Chen, Z., Sun, L., Klose, T., Pierson, T., Rossmann, M. and Kuhn, R. (2016). The 3.8Å resolution cryo-EM structure of Zika virus. DOI: 10.2210/pdb5ire/pdb Available at: http://www.rcsb.org/pdb/explore/explore.do?structureId=5IRE
One question that Jaenisch lab researchers have used their stem cell models to study is how the Zika virus affects brain development in a fetus. Zika is transmitted by mosquitoes, and when it infects a pregnant person, it can cause microcephaly or abnormally small head size, often accompanied by abnormal brain development and a range of related symptoms, in the fetus. Former Jaenisch lab postdocs Julien Muffat and Yun Li, and former graduate student in the lab Attya Omer Javed, collaborated with Lee Gehrke at the Massachusetts Institute of Technology (MIT) to understand how Zika affects brain cells. They turned stem cells into three cell types that play important roles in brain development: neural progenitors, astrocytes, and microglia. The researchers grew brain organoids or mini-brains with the cell types, and used this model of the brain to make several discoveries about how Zika affects brain development.
The same group of researchers, plus Heather Keys, the manager of Whitehead Institute’s Functional Genomics Platform, also grew neural progenitors from stem cells in order to identify which human genes the Zika virus relies on to infect its host. They then tested drugs known to affect the pathways they had identified, and found that these drugs protected the neural progenitors from Zika, providing good leads for possible medications.
Li, Muffat, and Omer Javed also used their brain organoid model to study a genetic mutation that can cause microcephaly. The mutation affects a cell division protein, KNL1. In collaboration with Whitehead Institute Member Iain Cheeseman, the researchers figured out how a mutation to a protein present throughout the body specifically affects brain cells during development.

Steven Lee/Whitehead Institute
Another brain-based developmental disorder that researchers in the Jaenisch lab study is Rett syndrome, a rare disorder that occurs primarily in girls and can cause severe impairments and developmental delays. There are currently no good treatments for Rett syndrome. The Jaenisch lab’s research seeks to better understand the mechanisms behind the disorder, as well as to find possible treatments for Rett syndrome.
In work led by former Jaenisch lab postdoc Xing Tang, the Jaenisch lab identified promising drug candidates that could help treat Rett syndrome. The researchers focused on an important neural gene, KCC2, that is implicated as contributing to Rett syndrome and developed a way to test whether drugs increased KCC2 expression in neurons. The screen identified a number of promising drug candidates, and because many of the drugs already have FDA approval for other uses, they could quickly be put to the test in clinical trials. The researchers hope that this approach could be used to speed up drug discovery for many brain disorders and diseases.

Steven Lee/Whitehead Institute
Whitehead Institute Member Richard Young’s lab has also done some research into Rett syndrome, collaborating with Jaenisch. In work led by former Young lab graduate students Charles Li and Eliot Coffey, researchers in the Young and Jaenisch labs determined that the main protein associated with Rett syndrome, MeCP2, typically forms condensates. Condensates are droplets that form when proteins and/or RNAs mesh together and separate out of their surroundings, like an oil droplet suspended in water. This allows the cell to organize where certain molecules gather. The researchers found that many of the mutations to MeCP2 that are associated with Rett syndrome disrupt its ability to form condensates. Jaenisch lab researchers continue to study the role of condensates in Rett syndrome. They hope that their findings may lead to new approaches for treating Rett syndrome that target condensates.

Immunofluorescence microscopy of MeCP2 neurons derived from mouse ESCs
Charles Li/ Whitehead Institute
Jaenisch lab postdoc Danielle Tomasello is also studying Rett syndrome. Her research is focused on astrocytes, a type of brain cell implicated in the disease. She is creating brain organoids that contain many different brain cell types in order to study the role of astrocytes in a rich, three-dimensional context that models the complexity of the brain.
Along with microcephaly and Rett syndrome, Jaenisch lab researchers have also studied fragile X syndrome, a developmental disorder that causes intellectual disability, primarily in males. Former postdoc Shawn Liu developed an approach that restores normal activity in neurons in mice with the genetic mutation that causes fragile X. The approach uses a modified version of CRISPR to turn genes off or on, rather than to edit the genetic code directly. The researchers hope it can be developed for use in people.
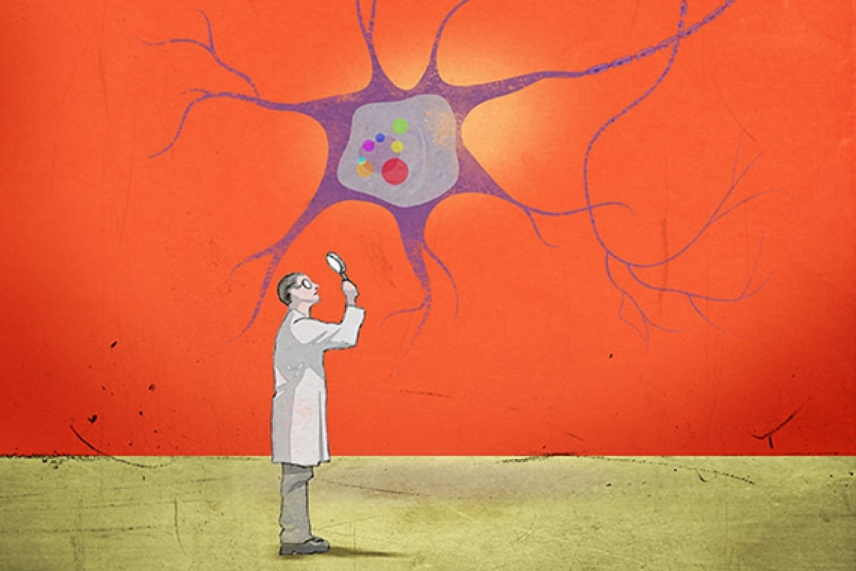
Steven Lee/Whitehead Institute
Research from the Jaenisch lab is also giving researchers a better understanding of a type of cancer that begins during fetal development. Neuroblastoma tumors originate in immature nerve cells and appear in the sympathetic nervous system, a system of nerves that runs through the body. The tumors are hard to study because they often appear during fetal development—when they are inaccessible—and shrink in infants, but then grow and become fatal in toddlers. Former postdoc in the Jaenisch lab Malkiel Cohen, collaborating with researchers from MIT’s Koch Institute and the Dana-Farber Cancer Institute, developed a chimeric mouse model—a mouse with human neural cells—in which to better study neuroblastoma. The researchers created neural crest cells with the relevant mutations, and implanted them into embryonic mice in order to observe how the tumors developed and evaded the immune system. The lab continues to research neuroblastomas with this model.
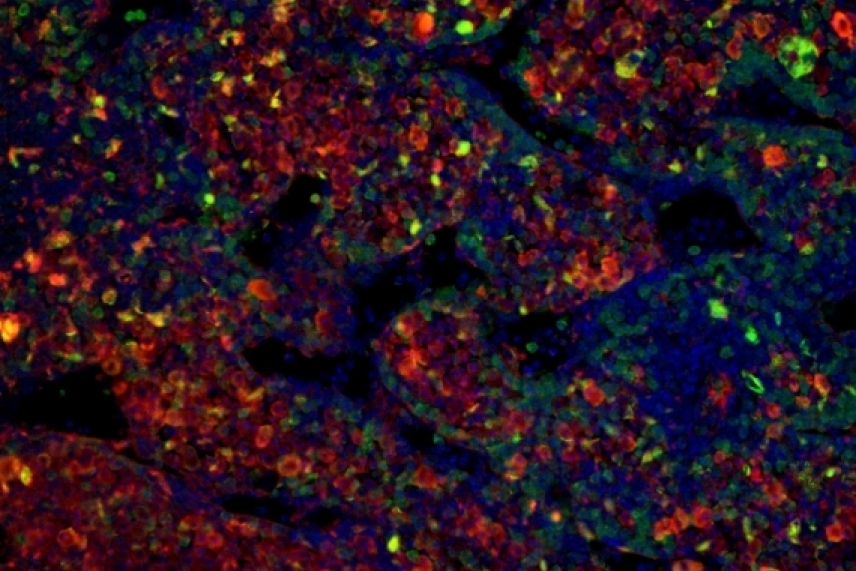
Malkiel Cohen/ Whitehead Institute
Through these projects, Jaenisch lab researchers are learning more about how the brain develops, what can go wrong during that development, and how we might be able to prevent or treat the disorders and diseases that cause brain development to go awry. The researchers also use their stem cell approaches to study other organs in the body and how these are affected in diseases from diabetes to fatty liver disease. They continue to perfect their recipes for creating mature cell types from stem cells, which will allow them to accurately model the human body past development and into adulthood.
Sex Chromosomes and Development
Sex differences–the effects of having different sex chromosomes–can greatly impact our development and health, and yet very little is known about the biology that drives these differences. Researchers in Whitehead Institute Member David Page’s lab aim to uncover this biology, and to raise awareness that other researchers should be considering the effects of sex chromosomes in their own areas of study. This will not only lead to a deeper understanding of human biology, but will have real impacts on medicine. Sex differences influence many aspects of health and disease in ways that are not yet understood: there are diseases that are much more likely to affect either men or women, yet no one knows what causes these differences. There are medicines that operate more or less well–or cause different side effects–in men versus women, yet historically, drug trials did not test for these differences. There are diseases and medical events such as heart attacks that can present differently in men versus women, and people may miss the signs in women because they have only been trained to look for the signs typical in men. Page’s hope is that as researchers learn more about the biology behind sex differences, and as everyone becomes more aware of the existence of such differences, this will make medicine both more equitable for populations whose biology has been understudied and more effective for everyone.
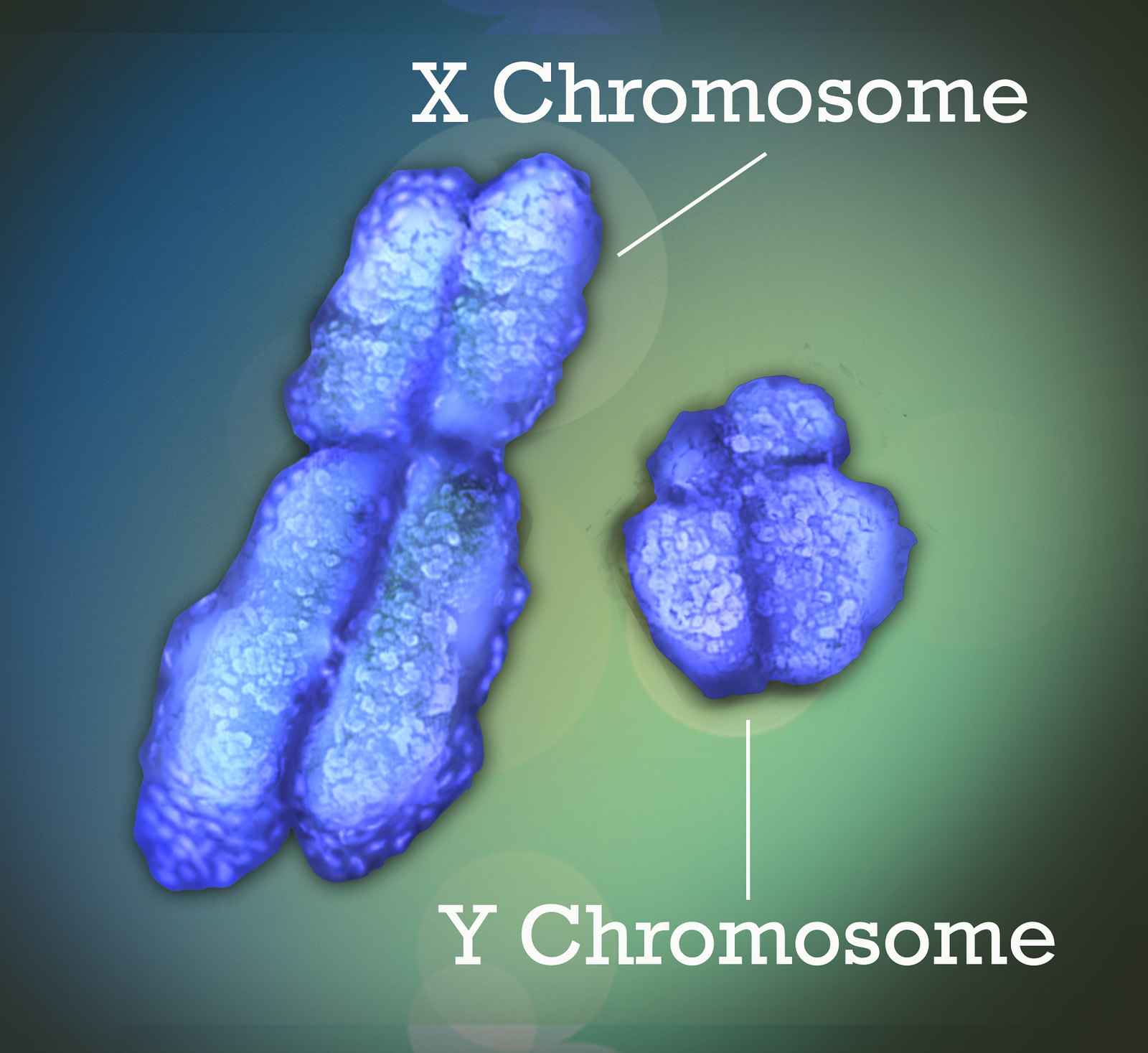
Page lab researchers study sex differences throughout the body. One of their areas of study is how having different sets of sex chromosomes can affect health, including during development. Most of us receive one sex chromosome from each parent: an X from our biological mother and an X or Y from our biological father. A gene on the Y chromosome, once activated, drives the development of male anatomy instead of female in an embryo. Activation of this gene kicks off a series of sex-based differences that will arise over the course of development. In other words, the activation of this one Y chromosome gene has a considerable impact on how an embryo develops. However, Page lab researchers are finding that the effects of having different sex chromosomes extend far beyond this single gene, and also beyond what can be explained by sex hormones. This is because the X and Y chromosomes contain genes unrelated to sex determination or hormones, these genes perform essential functions throughout the body, and there are differences between the X chromosome and Y chromosome versions of some of these genes. Therefore, having different sets of sex chromosomes leads to differences in gene expression in cells throughout body–not just in the reproductive tract.
Postdoc Adrianna San Roman studies the effects of having different sets of sex chromosomes on gene expression by mapping how gene expression corresponds to the number of each sex chromosome that a person has. In rare instances, people end up with different combinations of sex chromosomes than XX or XY. They can instead have anything from a single X chromosome (XO) to four X chromosomes (XXXX) to mixes such as XXY. San Roman uses samples from people with all of these different sex chromosome combinations in order to tease out connections between sex chromosome composition and gene expression, such as how expression of key genes changes with one X versus two versus four.
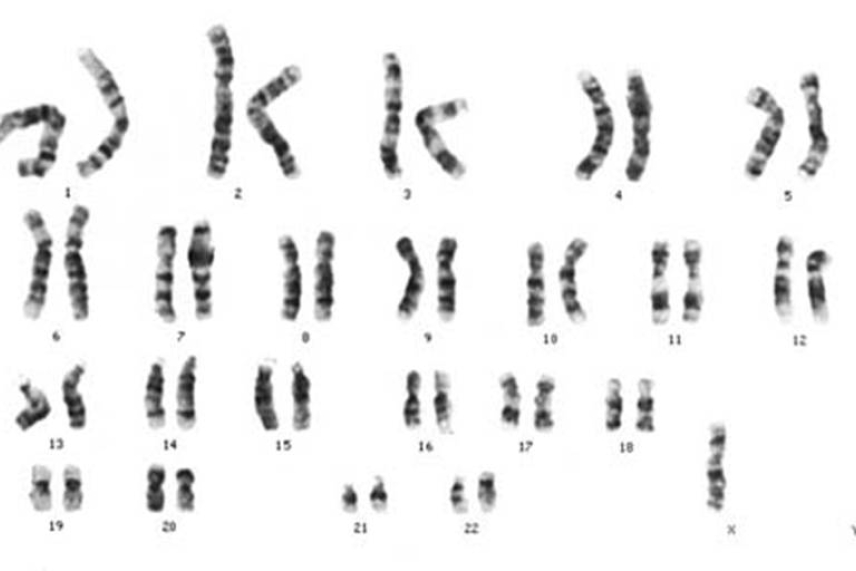
Karyotype with only one X chromosome and no Y chromosomes in the lower right. This is known as Turner Syndrome. Typically, a human male has one X and one Y chromosome and a female has two X chromosomes.
Wikimedia commons, licensed under CC BY-SA 3.0
San Roman hopes that her research can also prove useful to people with sex chromosome variations that can cause developmental and lifelong medical issues. Collecting samples for her work has connected her with communities such as that of women with Turner syndrome, which is caused by having XO sex chromosomes, and men with Klinefelter syndrome, which is caused by XXY chromosomes. Turner syndrome can cause developmental issues, delayed puberty, heart defects, infertility, and a number of other symptoms. Klinefelter syndrome can cause infertility, breast growth and breast cancer, osteoporosis, increased risk for a number of medical issues, and more. Page and San Roman hope that they and other researchers working on sex differences can combine forces with researchers working on these syndromes in order to find answers that are useful for all parties.
Page lab researchers also study questions of reproduction and development outside the focus of sex differences. Their work with sex cells has led the lab to some insights into cancers that originate during development. Former postdoc Peter Nicholls found that when germ cells—the cells that make eggs or sperm—fail to mature, and remain in a stem cell-like state, this can lead to testis cancer in male mice and (less frequently) tumors in the reproductive tract of female mice. Testis cancer is most commonly found in young boys, and understanding its cellular origins could help researchers develop treatments.

Primordial germ cells (red) are formed early in development and migrate to the gonad where they later undertake development to produce eggs or sperm.
Peter Nicholls/Whitehead Institute
Former Page lab postdoc Bluma Lesch and colleagues from the Dana Farber Cancer Institute, Broad Institute, and Harvard Medical School, meanwhile, found that genetic mutations in male mice can lead to higher cancer risk in their offspring—even when the mutation does not get passed on. They found that mutation to the gene Kdm6a in male mice increased the levels of methylation—the tags that turn genes on or off—in regions of their sperm. Usually, in mammals, epigenetic changes such as methylation tags are wiped clean early in embryonic development, but the researchers found that some of this extra methylation persisted in offspring of the Kdm6a-mutated mice. The offspring were then more likely to develop cancer in middle age and to die at a younger age. The researchers do not know if human embryos could inherit cancer risk in this same way—the resetting of epigenetic marks during human development could eliminate equivalent methylation—but they hope that further research may answer that question and, perhaps, shed light on how changes to a parent’s epigenome could affect offspring.
Building a foundation for medical advances
Whitehead Institute researchers continue to delve into the unknown aspects of development and developmental health. By building better models of human biology, they can observe what happens during development in human cells and organoids in the lab, instead of having to rely on animal models. By asking novel questions, and pursuing previously understudied topics like the role of sex chromosomes, they can gain a deeper understanding of the biology at hand. Their work is helping to uncover the intricacies of human development, and the myriad of ways in which small changes can cause this development to go awry. These findings may help to build a foundation of knowledge that researchers can draw upon to discover or design therapies that will address developmental disorders, diseases, and more.
Contact
Communications and Public Affairs
Phone: 617-452-4630
Email: newsroom@wi.mit.edu