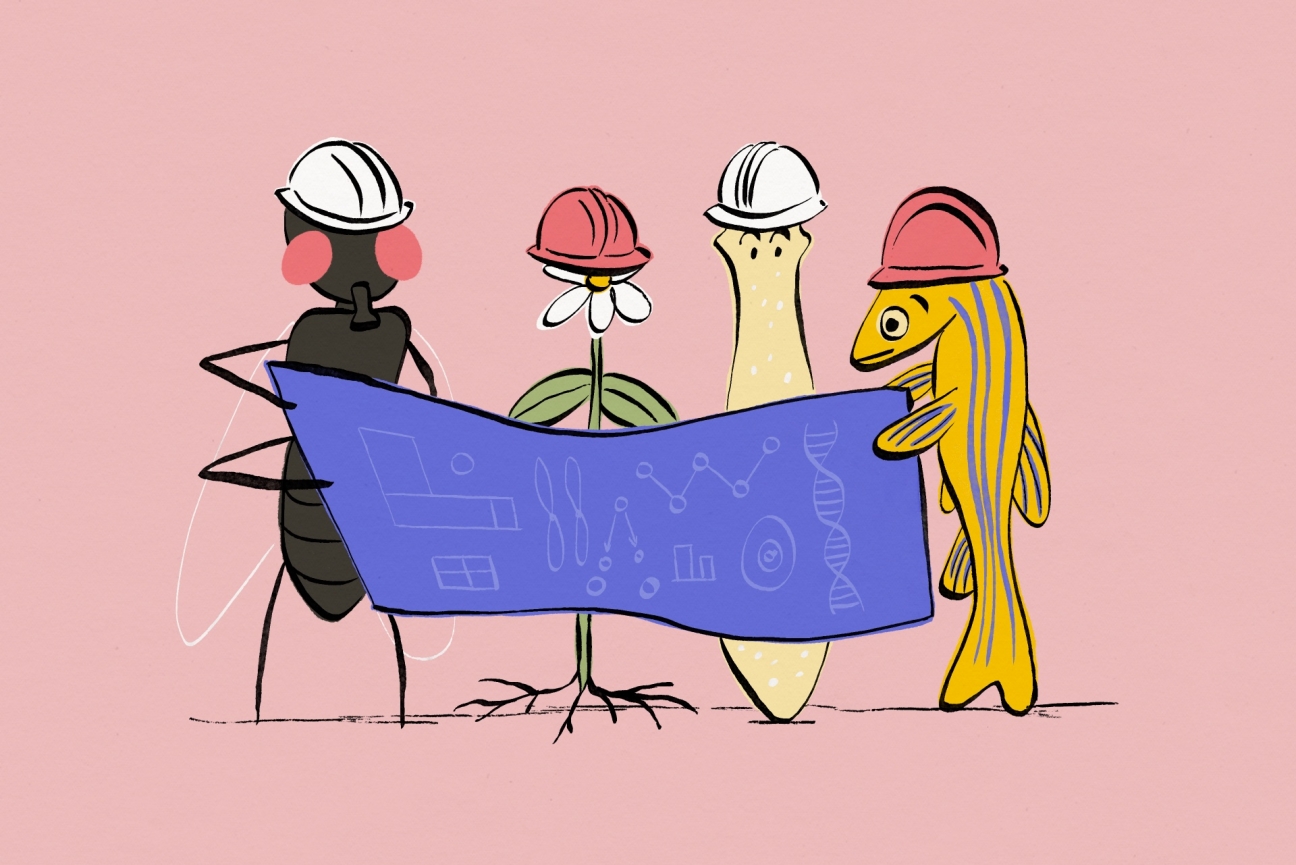
Daniel Gray-Barnett/Whitehead Institute
The blueprint of a body
This article is part of our Building a Body story collection. Read the entire collection here.
Multicellular organisms evolved over millennia into a dazzling array of differently adapted creatures. With each generation, tiny worms, lavishly plumed birds, and even humans must create themselves anew from a single cell. To do so, they require a plan.
“How that multifunctional body plan is created is one of the deepest questions in developmental biology,” said Zak Swartz, until recently a postdoctoral researcher in the lab of Whitehead Institute Member Iain Cheeseman. “How do you take a single cell and pattern into a body that has different functions and features along it?”
Whitehead Institute researchers are tackling this question through a variety of different lenses. Researchers in Iain Cheeseman’s lab, including Swartz, have delved into the mysterious forces that underlie the polarity of an organism’s first cell. For the lab led by Pulin Li, research comes in at a later stage of development, when multiple cells combine to form a tissue and must communicate with each other to become an organized whole. Work on regeneration in Peter Reddien’s lab shows how some creatures can access their body blueprint throughout their lives to repair nearly any injury, and Yukiko Yamashita’s group studies how organisms pass on their body blueprints to their offspring through germ cells. Jonathan Weissman and his lab have created a “map” which researchers can use to find the function of a given gene, allowing them access to an organism’s most fundamental plans. Read on to learn about these scientists' work, and more.
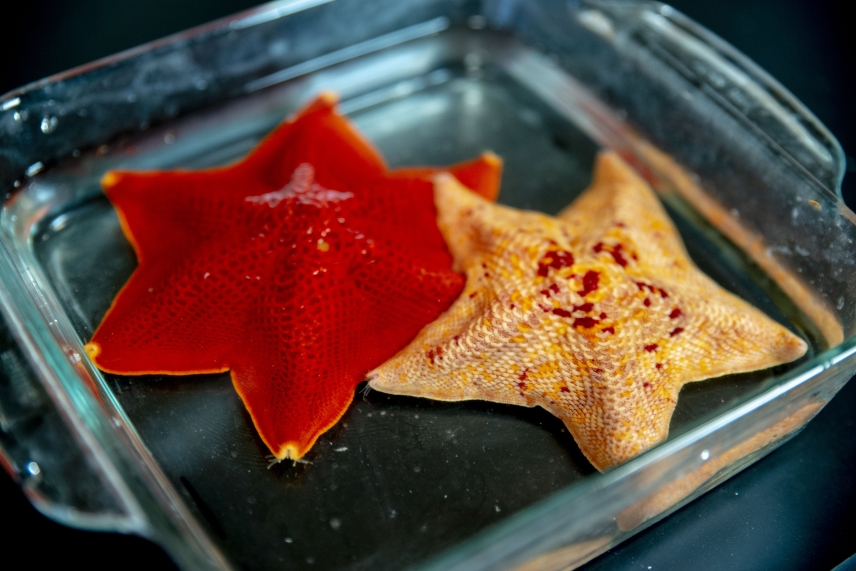
To study the intricate process of body patterning, Cheeseman Lab researchers used a type of sea star called the bat star, or Patiria miniata.
Conor Gearin/Whitehead Institute
Laying out the plan
All multicellular organisms begin with a single cell, the fertilized egg. This cell has an essential role in setting out the body plan for the rest of an organism. It all starts with establishing polarity — in other words, figuring out which side of the cell is the top, and which is the bottom. This polarity establishes an axis of symmetry for the growing organism, and sets the stage for other developmental processes to come.
In a 2021 study, Cheeseman and postdoctoral researcher Zak Swartz investigated how one protein in specific, called Disheveled, localizes in a cell to help create this polarity in sea star embryos. Swartz found that Disheveled started out uniformly distributed in small aggregations throughout the egg cell, or oocyte. As the cell prepared to divide, Disheveled aggregations dissolved and then reformed at what would become the “bottom” of the oocyte.
Once the initial polarity is established, the oocyte can divide, creating a bilaterally symmetric sea star larvae. The burgeoning cluster of cells must then undergo other processes to define the several axes of symmetry that adult sea stars are known for.
Pulin Li
Gretchen Ertl/Whitehead Institute
Talking through it
If an organism’s developmental blueprints are to be followed as development progresses, cells must be able to effectively communicate with each other. That cell to cell communication is the area of expertise of Whitehead Institute Member Pulin Li.
During her postdoctoral fellowship at the California Institute of Technology, Li studied tissue patterning — the mechanisms by which an organism’s newly forming tissues are laid out. Specifically, she investigated a developmental mechanism called morphogen gradient formation.
These gradients, composed of chemicals present in developing embryos, function as spatial coordinate systems and help determine how various cell types will be arranged in the organism — for example which groups of cells will form the liver, or the bones, or the brain, and where they will be within the body.
Li was able to recreate these gradients in the lab, in a Petri dish, and then interpret their signals using time lapse imaging and mathematical modeling. Here at Whitehead Institute, she follows a “bottom-up” approach to studying these complex systems. The best way to understand how something works, she says, is to build it yourself.
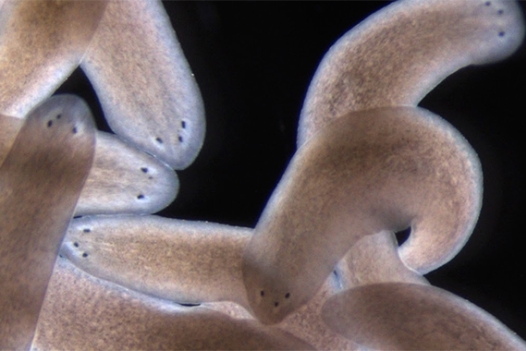
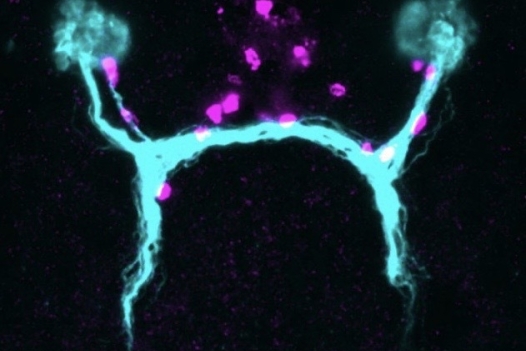
Fluorescent image of the visual system of the planarian Schmidtea mediterranea. Visual axons and photoreceptor neurons shown in cyan, guidepost-like cells shown in magenta.
Lucila Scimone/Whitehead Institute
The best laid plans…
As with any plan, there are times when things may go awry. Even when an organism is fully grown, there are times when the original “blueprints” may come in handy once again — especially when the organism is injured and needs to repair itself.
Most organisms have at least some tissues that can regenerate after injury, growing back nearly the same as before. And some organisms, such as a type of aquatic flatworm called a planarian, take regrowth a step further. Thanks to a special population of stem cells called neoblasts, planarians can regenerate an entire body from just a fraction of a worm. In fact, they can reproduce this way.
Whitehead Institute Member Peter Reddien studies the cells that give the worms this property. Beyond the shapeshifting neoblasts, planarians rely on muscle cells to set up a sort of global positioning system within the worms’ body. In case of an injury, these cells express signals that help stem cells orient themselves in the body and make the correct tissues.
Reddien’s lab has conducted experiments that revealed how an adult positioning system controls stem cells and the formation of new patterns of cells in regeneration — certain experiments, involving amputating a worm’s head in a manner that shifted the coordinates of the positioning system, tricked the system into regenerating a third eye.
They followed up on this nervous system regeneration in 2020, seeking to understand how exactly the axons — the “wires” that connect the eyes to the brain — were guided through the animals’ tissues.
They zeroed in on choice points, or places where the axon must “decide” which direction to go. In many organisms, these choice points are established early in development, but are not renewed when the animal reaches adulthood. In the planarians, Reddien and colleagues found that muscle cells were serving as choice points, or guideposts, for the growing axons, directing them toward the brain.
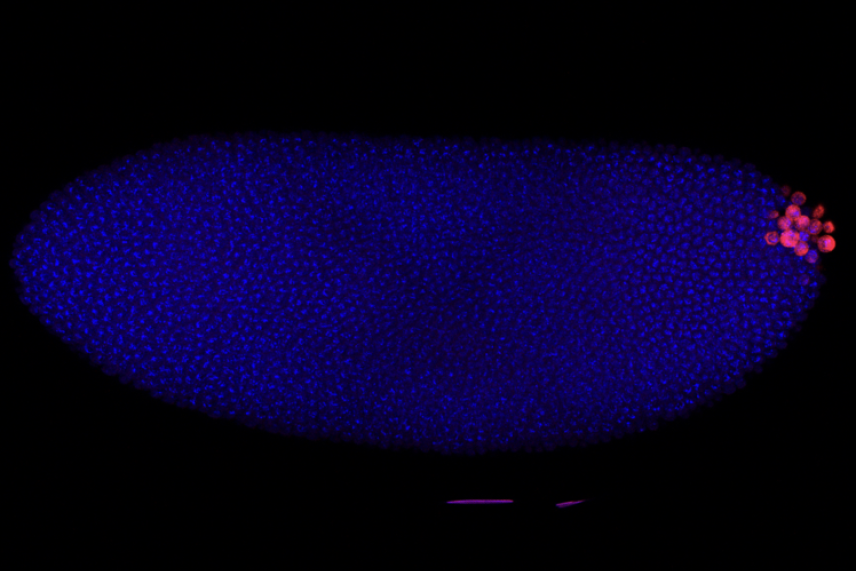
A Drosophila embryo with DNA stained in blue and germ cells stained in red. The germ cells are the first cells to form in a new embryo.
Ruoyu Chen/ Whitehead Institute
Passing on the blueprints
All cells in the body eventually die and decay when an organism dies — except for germ cells. The first cell of an organism, the fertilized egg, is a direct transfer from the parents that came before. In this way, cells of the germline stretch in an unbroken chain of life to our earliest ancestors.
“Germ cells never cease existing in the 1.5 video years of multicellular life,” said Whitehead Institute Member Yukiko Yamashita. “We knew that in a textbook way, but at some point in the last several years that really accurately hit our hearts. How are those cells not “going bad” over all that time? It seems impossible.”
In a new study, Yamashita and postdoctoral researcher George Watase laid out the mechanism by which the germline is able to bypass one of the hallmarks of cellular aging — a loss of ribosomal DNA. The study provides clues as to how germ cells are able to carry on the blueprints for life without losing their integrity over the millenia.
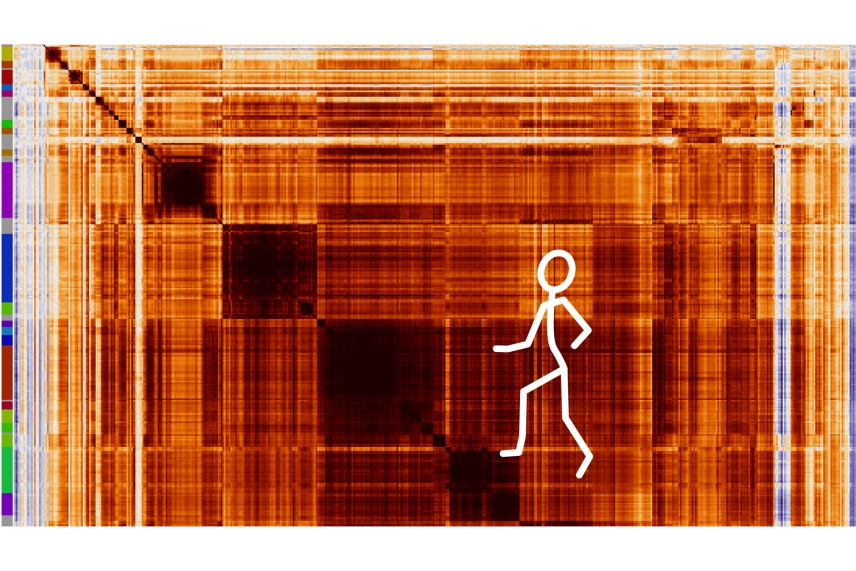
Whitehead Institute Member Jonathan Weissman and collaborators used their single-cell sequencing tool Perturb-seq on every expressed gene in the human genome, linking each to its job in the cell and creating a free resource for other researchers to address their own questions.
Jennifer Cook-Chrysos/ Whitehead Institute
Accessing the plans
All these various organismal plans are encoded in a creature’s DNA, and while scientists have sequenced the human genome in the past, many questions remain about the function of different genes. Whitehead Institute Member Jonathan Weissman used his lab’s newest genetic technology, a single-cell method called Perturb-Seq, to create a detailed map tying every expressed human gene to its function in the cell.
The Perturb-Seq method works by introducing small changes (or perturbations) into the genome, and then cataloging the RNAs produced in response to that genetic change. Their approach allows one to work at a massive scale making it possible to tie genotype to phenotype for every gene that is expressed. The map is available online for other researchers to use, and Weissman hopes that other researchers will take advantage of the tool for their own discovery-based research.
Looking ahead
From Cheeseman’s starfish to Yamashita’s flies to Reddien’s planarians, Whitehead Institute researchers continue to probe how different organisms design and execute their developmental plans in order to grow into complex creatures. Through their work, we are a step closer to understanding how the body blueprints that inform every creature on earth were drawn up by evolution, the oldest and most practiced architect.
Contact
Communications and Public Affairs
Phone: 617-452-4630
Email: newsroom@wi.mit.edu